Barking for Broccoli Uncovering the Growing Demand for Organic Dog Food
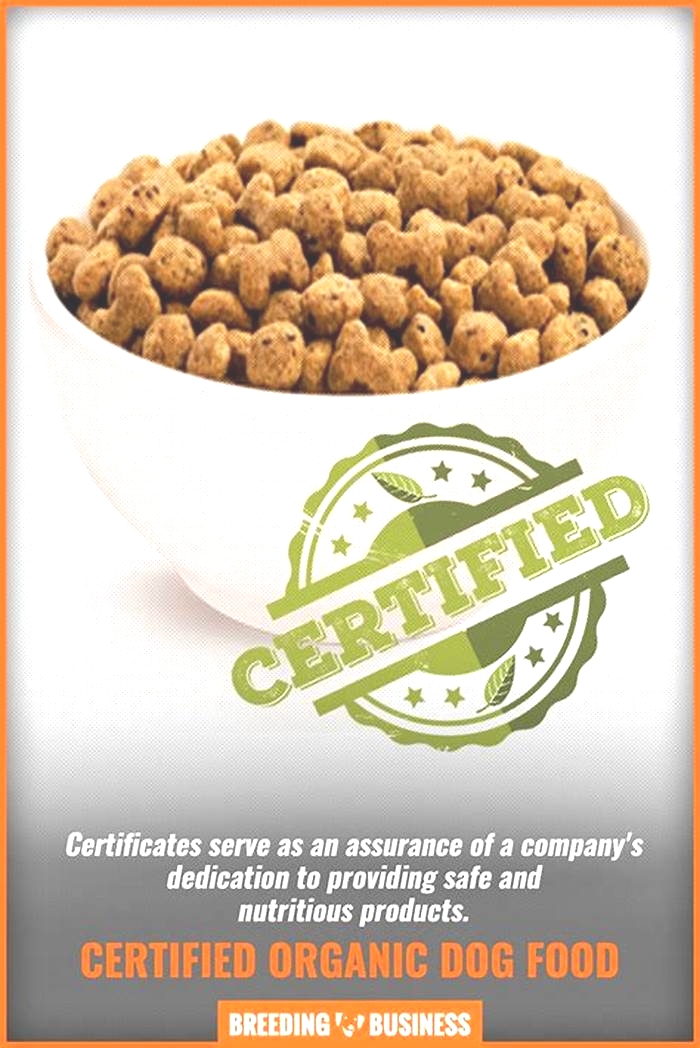
A meta-analysis of projected global food demand and population at risk of hunger for the period 20102050
Tilman, D., Balzer, C., Hill, J. & Befort, B. L. Global food demand and the sustainable intensification of agriculture. Proc. Natl Acad. Sci. USA 108, 20260 (2011).
Article ADS CAS PubMed PubMed Central Google Scholar
Alexandratos, N. & Bruinsma, J. World Agriculture Towards 2030/2050: The 2012 Revision (Food and Agriculture Organization of the United Nations, 2012).
Parry, M. L., Rosenzweig, C., Iglesias, A., Livermore, M. & Fischer, G. Effects of climate change on global food production under SRES emissions and socio-economic scenarios. Glob. Environ. Change 14, 5367 (2004).
Article Google Scholar
Hasegawa, T., Fujimori, S., Takahashi, K. & Masui, T. Scenarios for the risk of hunger in the twenty-first century using shared socioeconomic pathways. Environ. Res. Lett. 10, 014010 (2015).
Article ADS Google Scholar
Baldos, U. L. C. & Hertel, T. W. Debunking the new normal: why world food prices are expected to resume their long run downward trend. Glob. Food Sec. 8, 2738 (2016).
Article Google Scholar
Ishida, H. et al. Global-scale projection and its sensitivity analysis of the health burden attributable to childhood undernutrition under the latest scenario framework for climate change research. Environ. Res. Lett. 9, 064014 (2014).
Article ADS Google Scholar
Godfray, H. C. J. & Robinson, S. Contrasting approaches to projecting long-run global food security. Oxf. Rev. Econ. Policy 31, 2644 (2015).
Article Google Scholar
Reilly, M. & Willenbockel, D. Managing uncertainty: a review of food system scenario analysis and modelling. Phil. Trans. R. Soc. B 365, 30493063 (2010).
Article PubMed PubMed Central Google Scholar
van Dijk, M. & Meijerink, G. A review of global food security scenario and assessment studies: results, gaps and research priorities. Glob. Food Sec. 3, 227238 (2014).
Article Google Scholar
Huppmann, D., Rogelj, J., Kriegler, E., Krey, V. & Riahi, K. A new scenario resource for integrated 1.5C research. Nat. Clim. Change https://doi.org/10.1038/s41558-018-0317-4 (2018).
Headey, D. & Fan, S. Anatomy of a crisis: the causes and consequences of surging food prices. Agric. Econ. 39, 375391 (2008).
Article Google Scholar
Rosenzweig, C. et al. The Agricultural Model Intercomparison and Improvement Project (AgMIP): protocols and pilot studies. Agric. For. Meteorol. 170, 166182 (2013).
Article ADS Google Scholar
Bodirsky, B. L. et al. Global food demand scenarios for the 21st century. PLoS ONE 10, e0139201 (2015).
Article PubMed PubMed Central CAS Google Scholar
Robinson, S. et al. The International Model for Policy Analysis of Agricultural Commodities and Trade (IMPACT): Model Description for Version 3 (IFPRI, 2015); http://ssrn.com/abstract=2741234
Havlik, P. et al. Climate change mitigation through livestock system transitions. Proc. Natl Acad. Sci. USA 111, 37093714 (2014).
Article ADS CAS PubMed PubMed Central Google Scholar
Woltjer, G. et al. The MAGNET Model Module Description (Wageningen Economic Research, 2014).
Fujimori, S., Hasegawa, T. & Masui, T. in Post-2020 Climate Action (eds Fujimori, S. et al.) 305328 (Springer Singapore, 2017); https://doi.org/10.1007/978-981-10-3869-3_13
Stehfest, E., van Vuuren, D. P., Bouwman, L. & Kram, T. Integrated Assessment of Global Environmental Change with IMAGE 3.0: Model Description and Policy Applications (PBL Netherlands Environmental Assessment Agency, 2014).
Lotze-Campen, H. et al. Global food demand, productivity growth, and the scarcity of land and water resources: a spatially explicit mathematical programming approach. Agric. Econ. 39, 325338 (2008).
Google Scholar
Baldos, U. L. C. & Hertel, T. W. Looking back to move forward on model validation: insights from a global model of agricultural land use. Environ. Res. Lett. 8, 034024 (2013).
Article ADS Google Scholar
van der Mensbrugghe, D. The ENVironmental Impact and Sustainability Applied General Equilibrium (ENVISAGE) Model (World Bank, 2008).
Linehan, V. et al. Global food production and prices to 2050: scenario analysis under policy assumptions. In 43rd ABARES Outlook Conference (2013).
The Future of Food and AgricultureAlternative Pathways to 2050 (FAO, 2018).
FAO, IFAD, and WFP The State of Food Insecurity in the World: The Multiple Dimensions of Food Security 2013 (FAO, 2013).
Billen, G., Lassaletta, L., & Garnier, J. A vast range of opportunities for feeding the world in 2050: trade-off between diet, N contamination and international trade. Environ. Res. Lett. https://doi.org/10.1088/1748-9326/10/2/025001 (2015).
Msangi, S. & Batka, M. Major trends in diets and nutrition: a global perspective to 2050. In Frontiers of Economics and Globalization 227241 (Emerald Group Publishing, 2015).
Medek, D. E., Schwartz, J. & Myers, S. S. Estimated effects of future atmospheric CO2 concentrations on protein intake and the risk of protein deficiency by country and region. Environ. Health Perspect. 125, 8700187002 (2017).
Article Google Scholar
Shutes, L. et al. in Deliverable 7.4: Long-Term Supply, Food and Non-food Demand Drivers, Contrasting Scenarios and Their Impact on FNSa Report on Long-Term Supply, Food and Non-food Demand Drivers, Contrasting Scenarios and Their Impact on FNS Based on the Toolbox 2050 (ed. Shutes, L.) 332 (Wageningen Economic Research, 2017).
van Vuuren, D. P. et al. The Representative Concentration Pathways: an overview. Clim. Change 109, 531 (2011).
Article ADS Google Scholar
van Vuuren, D. P. et al. A new scenario framework for climate change research: scenario matrix architecture. Climatic Change 122, 373386 (2014).
Article Google Scholar
van Vuuren, D. P. et al. The shared socio-economic pathways: trajectories for human development and global environmental change. Glob. Environ. Change 42, 148152 (2017).
Article Google Scholar
ONeill, B. C. et al. The roads ahead: narratives for shared socioeconomic pathways describing world futures in the 21st century. Glob. Environ. Change 42, 169180 (2017).
Article Google Scholar
Willett, W. et al. Food in the Anthropocene: the EAT-Lancet commission on healthy diets from sustainable food systems. Lancet 393, 447492 (2019).
Article PubMed Google Scholar
Leclre, D. et al. Bending the curve of terrestrial biodiversity needs an integrated strategy. Nature 585, 551556 (2020).
Article ADS PubMed Google Scholar
FAO How to Feed the World in 2050 (High-Level Expert Forum, 2009).
Feed the world: a challenge and an opportunity. The John Deere Journal https://johndeerejournal.com/2015/12/smallholder-farmers-big-challenges (2015).
Carvajal-Yepes, M. et al. A global surveillance system for crop diseases. Science 364, 12371239 (2019).
Article ADS CAS PubMed Google Scholar
Holt-Gimnez, E. & Altieri, M. A. Agroecology, food sovereignty, and the new green revolution. Agroecol. Sustain. Food Syst. 37, 90102 (2013).
Google Scholar
Tomlinson, I. Doubling food production to feed the 9 billion: a critical perspective on a key discourse of food security in the UK. J. Rural Stud. 29, 8190 (2013).
Article Google Scholar
Claeys, P. Human Rights and the Food Sovereignty Movement: Reclaiming Control (Routledge, 2015).
Grethe, H., Dembele, A. & Duman, N. How to Feed the Worlds Growing Billions: Understanding FAO World Food Projections and Their Implications (Heinrich Bll Foundation and WWF Deutschland, 2011).
Hunter, M. C., Smith, R. G., Schipanski, M. E., Atwood, L. W. & Mortensen, D. A. Agriculture in 2050: recalibrating targets for sustainable intensification. BioScience 67, 386391 (2017).
Article Google Scholar
Kearney, J. Food consumption trends and drivers. Phil. Trans. R. Soc. B 365, 27932807 (2010).
Article PubMed PubMed Central Google Scholar
Wirsenius, S., Azar, C. & Berndes, G. How much land is needed for global food production under scenarios of dietary changes and livestock productivity increases in 2030? Agric. Syst. 103, 621638 (2010).
Article Google Scholar
vonLampe, M. et al. Why do global long-term scenarios for agriculture differ? An overview of the AgMIP global economic model intercomparison. Agric. Econ. 45, 320 (2014).
Article Google Scholar
Hertel, T. W. & Baldos, U. L. C. Attaining food and environmental security in an era of globalization. Glob. Environ. Change 41, 195205 (2016).
Article Google Scholar
Robinson, S. et al. Comparing supply-side specifications in models of global agriculture and the food system. Agric. Econ. 45, 2135 (2014).
Article Google Scholar
Valin, H. et al. The future of food demand: understanding differences in global economic models. Agric. Econ. 45, 5167 (2014).
Article Google Scholar
Risk of Hunger Pandemic as COVID-19 Set to Almost Double Acute Hunger by End of 2020 (World Food Programme, 2020); https://insight.wfp.org/covid-19-will-almost-double-people-in-acute-hunger-by-end-of-2020-59df0c4a8072
Gough, D., Oliver, S. & Thomas, J. (eds) An Introduction to Systematic Reviews (Sage, 2012); https://doi.org/10.1186/2046-4053-1-28
van Vuuren, D. P., Kok, M. T. J., Girod, B., Lucas, P. L. & de Vries, B. Scenarios in global environmental assessments: key characteristics and lessons for future use. Glob. Environ. Change 22, 884895 (2012).
Article Google Scholar
van Vuuren, D. P. & Carter, T. R. Climate and socio-economic scenarios for climate change research and assessment: reconciling the new with the old. Climatic Change 122, 415429 (2014).
Article ADS Google Scholar
Dellink, R., van der Mensbrugghe, D. & Saveyn, B. Shaping baseline scenarios of economic activity with CGE models: introduction to the special issue. J. Glob. Econ. Anal. 5, 127 (2020).
Article Google Scholar
Brjeson, L., Hjer, M., Dreborg, K.-H., Ekvall, T. & Finnveden, G. Scenario types and techniques: towards a users guide. Futures 38, 723739 (2006).
Article Google Scholar
Borenstein, M., Hedges, L. V., Higgins, J. P. T. & Rothstein, H. R. Introduction to Meta-analysis (John Wiley & Sons, 2009); https://doi.org/10.1002/9780470743386
Fox, J. & Weisberg, S. An R Companion to Applied Regression 3rd edn (Sage, 2019).
Bates, D., Mchler, M., Bolker, B. M. & Walker, S. C. Fitting linear mixed-effects models using lme4. J. Stat. Softw. 67, 148 (2015).
Article Google Scholar
Kuznetsova, A., Brockhoff, P. B. & Christensen, R. H. B. lmerTest package: tests in linear mixed effects models. J. Stat. Softw. https://doi.org/10.18637/jss.v082.i13 (2017).
Fox, J. & Weisberg, S. Visualizing fit and lack of fit in complex regression models with predictor effect plots and partial residuals. J. Stat. Softw. 87, 127 (2018).
Article Google Scholar
Diggle, P. J., Heagarty, P., Liang, K. Y. & Zeger, S. L. Analysis of Longitudinal Data 2nd edn (Oxford Univ. Press, 2002).
Zuur, A. F., Ieno, E. N., Walker, N., Saveliev, A. A. & Smith, G. M. Mixed Effects Models and Extensions in Ecology with R Statistics for Biology and Health (Springer, 2009); https://doi.org/10.1007/978-0-387-87458-6
Nelson, G. C. et al. Food Security, Farming, and Climate Change to 2050: Scenarios, Results, Policy Options (International Food Policy Research Institute, 2010); https://doi.org/10.2499/9780896291867
Pardey, P. G., Beddow, J. M., Hurley, T. M., Beatty, T. K. M. & Eidman, V. R. A bounds analysis of world food futures: global agriculture through to 2050. Aust. J. Agric. Res. Econ. 58, 571589 (2014).
Article Google Scholar
Popp, A. et al. Land-use futures in the Shared Socio-economic Pathways. Glob. Environ. Change 42, 331345 (2017).
Article Google Scholar
Riahi, K. et al. The Shared Socioeconomic Pathways and their energy, land use, and greenhouse gas emissions implications: an overview. Glob. Environ. Change 42, 153168 (2017).
Article Google Scholar
Stehfest, E. et al. Key determinants of global land-use projections. Nat. Commun. https://doi.org/10.1038/s41467-019-09945-w (2019).
Hasegawa, T. et al. Risk of increased food insecurity under stringent global climate change mitigation policy. Nat. Clim. Change 8, 699703 (2018).
Article ADS Google Scholar
Gouel, C. & Guimbard, H. Nutrition Transition and the Structure of Global Food Demand (CEPI, 2017).
Bijl, D. L. et al. A physically-based model of long-term food demand. Glob. Environ. Change 45, 4762 (2017).
Article Google Scholar
Food Security Indicators (FAO, 2020); http://www.fao.org/economic/ess/ess-fs/ess-fadata/en/#.XiYStoh7mcw
R Core Team R: A Language and Environment for Statistical Computing version 4.0.2 https://www.r-project.org/ (2021).
Dawson, T. P., Perryman, A. H. & Osborne, T. M. Modelling impacts of climate change on global food security. Clim. Change 134, 429440 (2016).
Article ADS Google Scholar
Rising Consumer Demand Reshapes Landscape for U.S. Organic Farmers
Feature: Organic Agriculture
Rising Consumer Demand Reshapes Landscape for U.S. Organic Farmers

Highlights:
Total organic-certified land in the United States has trended upward since 2000, reaching 4.89 million acres in 2021.
Regional differences in organic agriculture have become less pronounced. Organic acreage and sales historically concentrated in the Pacific region (particularly in California) have expanded across the country, most recently to the Appalachia and Delta regions.
As organic agriculture grows, organic producers have experienced increased market access. Conventional grocery retailers have overtaken natural food stores as the most popular outlet for organic food, with 55.6 percent of sales in 2021.
Authorized Federal funds to support organic research through USDA projects have increased in the last two decades. Mandatory spending authorization for the Organic Agriculture Research and Extension Initiative has grown from $3 million in 2002 to $50 million in 2023.
In the two decades since USDA published its final organic farming rules, the organic industry has experienced remarkable development. The higher prices producers generally receive for organic products compared with those grown under conventional methods (known as price premiums), motivated expanded U.S. organic production. Certified organic U.S. land for growing crops or livestock increased from 1.8 million in 2000 to 4.9 million in 2021. Organic sales in 2021 accounted for about 3 percent of U.S. farm receipts even though organic acreage was still less than 1 percent of U.S. farmland. Now, more regions are growing organic products, a wider range of retailers are selling organic food, and more research and development (R&D) funds are going toward development of organic agriculture.

Organic food and agriculture became a federally regulated industry in the United States after the USDA published the final rule in 2000 to implement the Organic Foods Production Act of 1990 (OFPA). Under the final rulewhich took full effect in 2002 USDA established the National Organic Program (NOP) to provide regulatory oversight so that all organic agricultural products sold, labeled, or represented as organic be in compliance with the regulations.
Initially, organic certified pasture and rangeland acreage grew faster than organic cropland, more than doubling between 2003 and 2004 from 750,000 acres to 1.59 million. U.S. pasture and rangeland primarily produceperennial grasses and legumes as forage for organically certified dairy cattle and other livestock. They also provide cover and food for wild game, songbirds, and other wildlife. Organically raised ruminant livestock, including cattle, sheep, and goats, must have access to organic pasture for the entire grazing period. By 2005, pasture and rangeland made up almost 60 percent of U.S. certified organic farmland at 2.33 million acres. Pasture and rangeland acreage has meandered since 2005, and the latest two USDA, National Agricultural Statistics Service (NASS) Organic Surveys (2019 and 2021) showed decreases.
Cattle raising for meat is the largest animal sector in U.S. agriculture, but it remains a small part of the organic animal products market. This is partly because of the time it took to implement an organic label for meat. The USDA, Food Safety and Inspection Service (FSIS) has jurisdiction over labeling of meat and poultry products under the Federal Meat Inspection Act and Poultry Products Inspection Act. Meanwhile, the Food and Drug Administration (FDA) has oversight authority over all other food labeling. Through the 1990s, FDA approved the use of organic labels for products under its purview, such as dairy. Meanwhile, FSIS did not allow for the use of the label on meat and poultry products until 1999. During that time, the imprecisely defined and unregulated natural label became popular. Competition with other labels, along with challenges such as following regulations for using organic feed, are potential reasons for declines in organic pasture and rangeland.
While organic pastureland surged in the early 2000s, cropland acreage grew slower, partly because it is more challenging to convert field crop production to organic farming systems. Organic pastureland and cropland both require producers to follow USDAs NOP standards that prohibit using substances such as most synthetic fertilizers and pesticides, thus restricting crop producers options for managing pests. Even so, rising consumer demand, especially for organic fruits and vegetables, has led to a steady increase in organic cropland acreage since 2000, with declines in just three NASS survey years (2002, 2014, and 2016).
Organic fruits and vegetables are the largest organic retail sales food category, surpassing $22 billion in 2022 and making up about 36 percent of all organic retail sales, according to the Organic Trade Association. Producers continually have increased the area devoted to organic specialty crops (the category for fruits, vegetables, and tree nuts). For example, fruit and nut acreage increased 76 percent between 2011 and 2021. With U.S. consumer demand for organic food growing faster than certified organic acreage, an extensive organic trade sector also emerged. In addition, organic cropland supports the organic animal industry by producing field crops such as corn and soybeans for animal feed.
Organic Production Shifts East
In the United States, the Pacific region (particularly California) historically has led in organic acreage and sales, accounting for about 43 percent of organic sales in 2012. Californias climate is well-suited for producing a variety of specialty crops (which includes fruits, vegetables, and tree nuts). The regions climate paired with early consumer interest in organics helped California blossom into the largest producer of organic products.
While California is the countrys largest producer of specialty crops, the Corn Belt is well-suited for field crops such as corn, soybeans, barley, and oats. These crops often are grown for animal feed or industrial uses, and the price benefit between them and conventional field crops often is not as high as it is for organic fruits and vegetables. Grain and oilseed growers have cited several barriers to transitioning from conventional production to organic methods. There is less technical assistance available for organic field crops than for conventional, the number of organic grain elevators is inadequate, and data on organic crop prices are limited. Additionally, unlike U.S. fruit and vegetable crops, most U.S. corn and soybean crops are grown with genetically engineered seed, a prohibited practice in NOP standards. Genetically engineered crops in neighboring operations, volunteer crops, or physical commingling of organic products with genetically engineered products all introduce the risk of low levels of genetically engineered material in organic crops. The risk of cross-pollination is low in soybeans, as the crop is self-pollinated, but is higher for cross-pollinated crops such as corn.
In recent years, regional differences in organic agriculture have become less pronounced as acreage and sales across the country have increased. The Pacific region still accounted for about 32 percent of sales in 2021, but shares of sales have increased in most other regions. For instance, organic sales in the Corn Belt accounted for 8 percent of sales in 2021, up from about 6 percent in 2012. In the Northeast, the share of organic sales rose to almost 16 percent from almost 11 percent in 2012. Regions that historically accounted for a lower share of organic salessuch as the Corn Belt, Delta, Southeast and Southern Plainsall saw gains from 2012 to 2021, including a 3,582-percent increase in the Delta, where organic sales rose from $4 million to $143 million.
Conventional Grocers, Online Vendors Gain Strength in Organic Food Retailing
As demand for and production of organic agriculture grew, organic products found increased market access. From the 1970s, when organic markets emerged in the United States, until the mid-2000s, most organic retail sales occurred in natural food stores. Conventional grocery retailers have since become the most popular outlet for organic food, with 55.6 percent of sales in 2021. Producers also continue to market their products directly to consumers, via farmers markets, Community Supported Agriculture programs, farm co-ops, and the internet. The share of direct-to-consumer sales peaked at 12.6 percent in 2021 after stalling at 10 percent from 2006 to 2012.
While sales through offline direct-to-consumer channels have decreased since 2010, growers have expanded their use of the internet in marketing their products directly to consumers, particularly during the Coronavirus (COVID-19) pandemic. In 2012, internet sales accounted for 2.1 percent of total organic sales, increasing to 4.5 percent by 2019 and 6.5 percent in 2021. These percentages include items ordered from online stores and shipped to consumers but do not include groceries ordered online then picked up by consumers or third parties (such as InstaCart) and, in some cases, when stores deliver to homes. Use of retail options differs by State as well. For example, the NASS Organic Survey shows that California producers sell 20 percent of their products directly to consumers, but Indiana growers market only 1 percent directly.
Research and Development Funds
Investment in research and innovation is critical for continued growth in the organic sector. Generally, private and public research play complementary roles, with the private sector concentrating on areas with commercial application and public research focusing on natural resources, human nutrition, and areas of potential societal benefits. Much of the industry research on organic agriculture has concentrated on biological pest control products, such as natural enemies or botanical extracts, and development of new food products. Given the environmental benefits of organic agriculture, public research is extensive. Research on production, breeding, processing, and marketing of commodities can help producers transition into organic agriculture by reducing uncertainties.
In a 1980 report assessing the nature and activity of organic agriculture, USDA recognized the lack of necessary information available to organic and transitioning farms, noting limited vital statistics (such as the number of farms and geographic distribution) and Government- and university-funded research. In 1995, only 0.1 percent of USDA research projects were explicitly related to organic farming. The 1998 Agricultural Research, Extension, and Education Reform Act (1998 Farm Act) began to address the information gap by setting priorities on facilitating organic development and improvement, evaluating its economic benefits, and exploring international trade opportunities.
The 1998 Farm Act created the Organic Transitions Program (ORG) as a competitive grants program under USDAs National Institute of Food and Agriculture (NIFA) to support research on the competitiveness of organic producers and on overcoming barriers to transition. The 2002 Farm Act launched the NIFA Organic Agriculture Research and Extension Initiative (OREI), a competitive grants program intended to make it easier for producers to grow and market organic products. Initial priorities for OREI included determining desirable traits of organic commodities, identifying constraints for expansion, and conducting on-farm research. The 2008 Farm Act added priorities on studying the relationship between organic systems and conservation, organic systems and environmental outcomes, and development of new seed varieties.
ORG originally was funded at $500,000 annually, although its funding is not included in the Farm Bill and is typically lower than that of NIFAs OREI program. The initial mandatory spending authorization for OREI was set at $3 million a year but has grown throughout the decades and is now set at $50 million a year. Grant payments are always less than the authorized amount because of automatic Federal spending reductions and costs incurred by the program such as those for administrative needs. In 2020, the number of proposals received since the programs began exceeded 1,700, but only 356 were funded.
OREI does not offer direct support to farmers but provides indirect benefits through research and by imparting knowledge. Other USDA agencies have programs directly assisting organic producers. Since 2008, the Farm Acts have included provisions to reduce obstacles organic farmers face in trying to access USDA farm assistance programs. Programs have become more tailored to organic production, and USDA has increased its outreach to organic producers. USDA recently created the Organic Transition Initiative (OTI) with $300 million to provide comprehensive support for farmers transitioning to organic production and to support established organic producers. OTI helps growers overcome barriers to transition, including a lack of region-specific and technical knowledge, price and yield risk, and market access.

This article is drawn from...
U.S. Organic Production, Markets, Consumers, and Policy, 200021, by Andrea Carlson, Catherine Greene, Sharon Raszap Skorbiansky, Claudia Hitaj, Kim A. Ha, Michel Cavigelli, Peyton Ferrier, and William D. McBride, ERS, March 2023
You may also be interested in...
Organic Agriculture, by Sharon Raszap Skorbiansky, USDA, Economic Research Service, January 2024
Organic Mushroom Industry Expands To Meet Rising Consumer Interest, by Catharine Weber and Sharon Raszap Skorbiansky, USDA, Economic Research Service, July 2023
Organic Mushroom Production Concentrated in Pennsylvania, by Sharon Raszap Skorbiansky, USDA, Economic Research Service, October 2023
With Expanded Options, Organic Producers of Specialty Crops Increase Use of Federal Risk Management Products, by Gregory Astill and Sharon Raszap Skorbiansky, USDA, Economic Research Service, October 2023
Prevalence of the Natural Label Varies by Food Category, by Fred Kuchler and Megan Sweitzer, USDA, Economic Research Service, August 2023