What are the potential dangers of organic foods
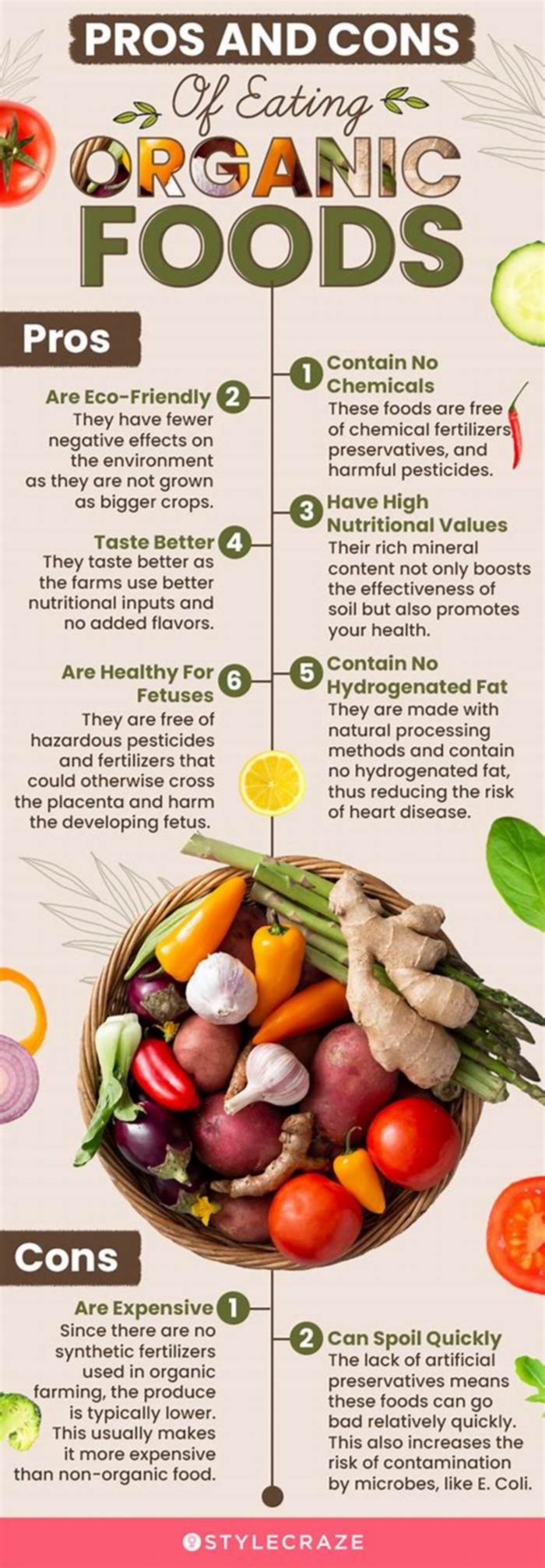
Human health implications of organic food and organic agriculture: a comprehensive review
Background
The long-term goal of developing sustainable food systems is considered a high priority by several intergovernmental organisations [13]. Different agricultural management systems may have an impact on the sustainability of food systems, as they may affect human health as well as animal wellbeing, food security and environmental sustainability. In this paper, we review the available evidence on links between farming system (conventional vs organic) and human health.
Food production methods are not always easy to classify. This complexity stems from not only the number and varying forms of conventional and organic agricultural systems but also resulting from the overlap of these systems. In this paper, we use the term conventional agriculture as the predominant type of intensive agriculture in the European Union (EU), typicallywith high inputs of synthetic pesticides and mineral fertilisers, and a high proportion of conventionally-produced concentrate feed in animal production. Conversely,organic agriculture is in accordance with EU regulations or similar standards for organic production, comprising the use of organic fertilisers such as farmyard and green manure, a predominant reliance on ecosystem services and non-chemical measures for pest prevention and control and livestock access to open air and roughage feed.
In 2015, over 50.9 million hectares, in 179 countries around the world, were cultivated organically, including areas in conversion [4]. The area under organic management (fully converted and in-conversion) has increased during the last decades in the European Union, where binding standards for organic production have been developed [5, 6]. In the 28 countries forming the EU today, the fraction of organically cultivated land of total agricultural area has been steadily increasing over the last three decades.0.1%, 0.6%, 3.6%, and 6.2% of agricultural land were organicin 1985, 1995, 2005, and 2015,respectively, equalling 11.2 million ha in 2015 [79]. In 7 EU Member States, at least 10% of the agricultural land is organic [7]. In 2003, 125,000 farms in the EU were active in organic agriculture, a number that increased to 185,000 in 2013 [10]. Between2006and2015, the organic retail market has grown by 107% in the EU, to 27.1 billion [7].
This review details the science on the effects of organic food and organic food production on human health and includes
studies that directly address such effects in epidemiological studies and clinical trials.
animal and in vitro studies that evaluate biological effects of organic compared to conventional feed and food.
Focusing on narrower aspects of production, we then discuss the impact of the production system on
(3)
plant protection, pesticide exposure, and effects of pesticides on human health,
(4)
plant nutrition, the composition of crops and the relevance for human health,
(5)
animal feeding regimens, effects on the composition of animal foods and the relevance for human health.
(6)
animal health and well-being, the use of antibiotics in animal production, its role in the development of antibiotic resistance, and consequences of antibiotic resistance for public health.
In the discussion, we widen the perspective from production system to food system and sustainable diets and address the interplay of agricultural production system and individual food choices. The consequences of these aspects on public health are briefly discussed.
Due to a limited evidence base, minimal importance, lack of a plausible link between production system and health, or due to lack of relevance in the European Union, we do not or only briefly touch upon
singular food safety events such as outbreaks of diseases that are not clearly caused by the production system (hygiene regulations for plant production and for animal slaughtering and processing are for the most part identical for organic and conventional agriculture) or fraudulent introduction of contaminated feed into the feed market
historic events and historic sources of exposure, such as the BSE crisis caused by the now-banned practice of feeding cattle with meat and bone meal from cattle, or continuing effects of the historic use of DDT, now banned in all agricultural contexts globally
contaminants from food packaging
aspects of food processing, such as food additives
the presence of mycotoxins in consequence of post-harvest storage and processing which is governed chiefly by moisture and temperature in storage
the use of growth hormones in animal production, which is not permitted in the EU but in several other countries
Furthermore, aspects of environmental sustainability, such as biodiversity and greenhouse gas emissions, may also be affected by the agricultural production system [11, 12] and may affect human health via food security [13, 14].While theseindirect links are outside the scope of this review, we briefly touchon them in the discussion. Also, the focus of this article is on public health, not on occupational health of agricultural workers or local residents, although these issues are considered as part of the epidemiological evidence on pesticide effects. While agricultural standards vary between countries and regions, we maintain a global perspective when appropriate and otherwise focus on the European perspective.
The literature search for this review was carried out at first using the PubMed and Web of Science databases, while applying organic food or organic agriculture along with the most relevant keywords, through to the end of 2016 (more recent references were included, when relevant, although they were not identified through the systematic search). We made use of existing systematic reviews and meta-analyses when possible. In some cases, where scientific literature is scarce, we included grey literature e.g. from authorities and intergovernmental organisations. We also considered references cited in the sources located.
Association between organic food consumption and health: Findings from human studies
A growing literature is aiming at characterizing individual lifestyles, motivations and dietary patterns in regard to organic food consumption, which is generally defined from responses obtained from food frequency questionnaires [1523]. Still, current research on the role of organic food consumption in human health is scarce, as compared to other nutritional epidemiology topics. In particular, long-term interventional studies aiming to identify potential links between organic food consumption and health are lacking, mainly due to high costs. Prospective cohort studies constitute a feasible way of examining such relationships, although compliance assessment is challenging. Considering a lack of biomarkers of exposure, the evaluation of the exposure, i.e. organic food consumption, will necessarily be based on self-reported data that may be prone to measurement error.
Some recent reviews have compiled the findings [2426] from clinical studies addressing the association between consumption of organic food and health. These studies are scant and generally based on very small populations and short durations, thus limiting statistical power and the possibility to identify long-term effects. Smith-Spangler et al. [25] summarised the evidence from clinical studies that overall no clinically significant differences in biomarkers related to health or to nutritional status between participants consuming organic food compared to controls consuming conventional food. Among studies of nutrient intakes, the OrgTrace cross-over intervention study of 33 males, the plant-based fraction of the diets was produced in controlled field trials, but 12days of intervention did not reveal any effect of the production system on the overall intake or bioavailability of zinc and copper, or plasma status of carotenoids [27, 28].
In observational studies, a specific challenge is the fact that consumers who regularly buy organic food tend to choose more vegetables, fruit, wholegrain products and less meat, and tend to have overall healthier dietary patterns [18, 29]. Each of these dietary characteristics is associated with a decreased risk for mortality from or incidence of certain chronic diseases [3036]. Consumers who regularly buy organic food are also more physically active and less likely to smoke [18, 19, 37]. Depending on the outcome of interest, associations between organic vs conventional food consumption and health outcome therefore need to be carefully adjusted for differences in dietary quality and lifestyle factors, and the likely presence of residual confounding needs to be considered. In children, several studies have reported a lower prevalence of allergy and/or atopic disease in families with a lifestyle comprising the preference of organic food [3844]. However, organic food consumption is part of a broader lifestyle in most of these studies and associated with other lifestyle factors. Thus, in the Koala birth cohort of 2700 mothers and babies from the Netherlands [39], exclusive consumption of organic dairy products during pregnancy and during infancy was associated with a 36% reduction in the risk of eczema at age 2years. In this cohort, the preference of organic food was associated with a higher content of ruminant fatty acids in breast milk [40], which in turn was associated with a lower odds ratio for parent-reported eczema until age 2y [45].
In the MOBA birth cohort study of 28,000 mothers and their offspring, women reporting a frequent consumption of organic vegetables during pregnancy exhibited a reduction in risk of pre-eclampsia [29] (OR=0.79, 95% CI 0.62 to 0.99). No significant association was observed for overall organic food consumption, or five other food groups, and pre-eclampsia.
The first prospective study investigating weight change over time according to the level of organic food consumption included 62,000 participants of the NutriNet-Sant study. BMI increase over time was lower among high consumers of organic food compared to low consumers (mean difference as % of baseline BMI= 0.16, 95% Confidence Interval (CI): 0.32; 0.01). A 31% (95%CI: 18%; 42%) reduction in risk of obesity was observed among high consumers of organic food compared to low consumers. Two separate strategies were chosen to properly adjust for confounders [46]. This paper thus confirms earlier cross-sectional analyses from the same study [18].
In regardto chronic diseases, the number of studies is limited. In the Nutrinet-Sant study, organic food consumers (occasional and regular), as compared to non-consumers, exhibited a lower incidence of hypertension, type 2 diabetes, hypercholesterolemia (in both males and females), and cardiovascular disease (in men) [47] but more frequently declared a history of cancer. Inherent to cross-sectional studies, reverse causation cannot be excluded; for example, a cancer diagnosis by itself may lead to positive dietary changes [48].
Only one prospective cohort study conducted in adults addressed the effect of organic food consumption on cancer incidence. Among 623,080 middle-aged UK women, the association between organic food consumption and the risk of cancer was estimated during a follow-up period of 9.3 y. Participants reported their organic food consumption through a frequency question as never, sometimes, or usually/always. The overall risk of cancer was not associated with organic food consumption, but a significant reduction in risk of non-Hodgkin lymphoma was observed in participants who usually/always consume organic food compared to people who never consume organic food (RR=0.79, 95%CI: 0.65; 0.96) [37].
In conclusion, the link between organic food consumption and health remains insufficiently documented in epidemiological studies. Thus, well-designed studies characterized by prospective design, long-term duration and sufficient sample size permitting high statistical power are needed. These must include detailed and accurate data especially for exposure assessment concerning dietary consumption and sources (i.e. conventional or organic).
Experimental in vitro and animal studies
In vitro studies
The focus on single plant components in the comparison of crops from organic and conventional production, as discussed further below, disregards the fact that compounds in food do not exist and act separately, but in their natural context [49]. In vitro studies of effects of entire foods in biological systems such as cell lines can therefore potentially point at effects that cannot be predicted from chemical analyses of foods, although a limitation is that most cells in humans are not in direct contact with food or food extracts.
Two studies have investigated the effect of organic and conventional crop cultivation on cancer cell lines, both using crops produced under well-documented agricultural practices and with several agricultural and biological replicates. In the first study extracts from organically grown strawberries exhibited stronger antiproliferative activity against one colon and one breast cancer cell line, compared to the conventionally produced strawberries [50]. In the second study [51] the extracts of organic naturally fermented beetroot juices induced lower levels of early apoptosis and higher levels of late apoptosis and necrosis in a gastric cancer cell line, compared to the conventional extracts. Both studies thus demonstrated notable differences in the biological activity of organic vs. conventionally produced crop extracts in vitro, which should inspire further research. However, neither of these studies allows for the distinction of a selective antiproliferative effect on cancer cells, and general cell toxicity. Therefore it cannot be determined which of the organic or conventional food extracts, if any, had the preferable biological activity in terms of human health.
Animal studies of health effects
Considering the difficulties of performing long-term dietary intervention studies in humans, animal studies offer some potential of studying long-term health effects of foods in vivo. However, extrapolation of the results from animal studies to humans is not straight-forward. Studies in this field started almost 100years ago.A review of a large number of studies [52] concluded that positive effects of organic feed on animal health are possible, but further research is necessary to confirm these findings. Here we focus on the main health aspects.
In one of the best-designed animal studies, the second generation chickens receiving the conventionally grown feed demonstrated a faster growth rate. However, after an immune challenge, chickens receiving organic feed recovered more quickly [53]. This resistance to the challenge has been interpreted as a sign of better health [54, 55].
In one carefully conducted crop production experiment, followed by a rat feeding trial, the production system had an apparent effect on plasma-IgG concentrations but not on other markers of nutritional or immune status [56]. A two-generational rat study based on feed grown in a factorial design (fertilisation x plant protection) of organic and conventional practices revealed that the production system had an effect on several physiological, endocrine and immune parameters in the offspring [57]. Most of the effects identified were related to the fertilisation regimen. None of these studies foundthat any of the feed production systems was more supportive of animal health.
Several other studies, mostly in rats, have reported some effect of the feed production system on immune system parameters [5760]. However, the direct relevance of these findings for human health is uncertain.
Collectively, in vitro and animal studies have demonstrated that the crop production system does have an impact on certain aspects of cell life, the immune system, and overall growth and development. However, the direct relevance of these findings for human health is unclear. On the other hand, these studies may provide plausibility to potential effects of conventional and organic foods on human health. Still, most of the outcomes observed in animal studies have not been examined in humans so far.
Pesticides
Plant protection in organic and conventional agriculture
Plant protection in conventional agriculture is largely dependent on the use of synthetic pesticides.Conversely, organic farming generally relies on prevention and biological means for plant protection, such as crop rotation, intercropping, resistant varieties, biological control employing natural enemies, hygiene practices and other measures [6164]. Yet, certain pesticides are approved for use in organic agriculture. In the EU, pesticides (in this context, more specifically chemical plant-protection products; micro- and macrobiological agents are excluded from this discussion due to their low relevance for human health) are approved after an extensive evaluation, including a range of toxicological tests in animal studies [65]. Acceptable residue concentrations in food are calculated from the same documentation and from the expected concentrations in accordance with approved uses of the pesticides. Currently, 385 substances are authorised as pesticides in the EU (Table). Of these, 26 are also approved for use in organic agriculture [6, 66] as evaluated in accordance with the same legal framework.
Table 1
Active substances approved in the EU and important toxicological properties according to risk assessments by EFSA. Data compiled from the EU pesticides database [66] and from Commission Regulation 889/2008 (consolidated version 201611-07) Annex II Sections 13 [6]
Approved in EU agriculturea | Also approved in EU organic agriculturea | |
---|---|---|
Total number of EU-approved active substances (+ basic substancesb) | 385 (+15) | 26 (+10) |
Of these: | ||
Any identified toxicityc | 340 | 10 |
Classified asd | ||
Acutely toxic class 1+2+3+4, totale | 5+17+26+76, 99 | 0+0+2+2, 3f |
Carcinogenicity category 2g | 27 | 0 |
Germ cell mutagenicity category 2h | 2 | 0 |
Reproductive toxicity category 1B+2i | 5+21 | 0 |
Candidate for substitutionj | ||
Low ADI/ARfD/AOEL | 19 | 0 |
Two PBT criteria fulfilledk | 54 | 1l |
Reproductive toxicity 1Bi | 5 | 0 |
Endocrine disrupting properties | 5 | 0 |
Most of the pesticides approved for organic agriculture are of comparatively low toxicological concern for consumers because they are not associated with any identified toxicity (e.g. spearmint oil, quartz sand), because they are part of a normal diet or constitute human nutrients (e.g. iron, potassium bicarbonate, rapeseed oil) or because they are approved for use in insect traps only and therefore have a negligible risk of entering the food chain (i.e. the synthetic pyrethroids lambda-cyhalothrin and deltamethrin, and pheromones). Two notable exceptions are the pyrethrins and copper. Pyrethrins, a plant extract from Chrysanthemum cinerariaefolium, share the same mechanism of action as the synthetic pyrethroid insecticides, but are less stable. Copper is an essential nutrient for plants, animals and humans, although toxic at high intakes and of ecotoxicological concern due to toxicity to aquatic organisms.
Plant protection practices developed in and for organic agriculture may be of benefit to the entire agricultural system [6770]. This is of specific value for the transition towards sustainable use of pesticides in the EU, which has a strong emphasis on non-chemical plant protection measures including prevention and biological agents [63, 64]. Further, steam treatment of cereal seeds for the prevention of fungal diseases (http://thermoseed.se/) has been developed driven by the needs of organic agriculture as an alternative to chemical seed treatments [71, 72]. These methods are now also being marketed for conventional agriculture, specifically for integrated pest management (IPM) [73].
Pesticide use Exposure of consumers and producers
One main advantage of organic food production is the restricted use of synthetic pesticides [5, 6], which leads to low residue levels in foods and thus lower pesticide exposure for consumers. It also reduces the occupational exposure of farm workers to pesticides and drift exposures of rural populations. On average over the last three available years, EFSA reports pesticide residues below Maximum Residue Levels (MRL) in 43.7% of all and 13.8% of organic food samples. MRLs reflect the approved use of a pesticide rather than the toxicological relevance of the residue. There are no separate MRLs for organic products. A total of 2.8% of all and 0.9% of organic samples exceeded the MRL, which may be due to high residue levels or due to low levels but unapproved use of a particular pesticide on a particular crop [7476]. Of higher toxicological relevance are risk assessments, i.e. expected exposure in relation to toxicological reference values. On average 1.5% of the samples were calculated to exceed the acute reference dose (ARfD) for any of the considered dietary scenarios, with the organophosphate chlorpyrifos accounting for approximately half of these cases and azole fungicides (imazalil, prochloraz, and thiabendazole) for approximately 15%. None (0%) of the organic samples exceeded the ARfD [74]. Residues of more than one pesticide were found in approximately 25% of the samples but calculations of cumulative risks were not included in the reports [7476].
The only cumulative chronic risk assessment comparing organic and conventional products known to us has been performed in Sweden. Using the hazard index (HI) method [77], adults consuming 500g of fruit, vegetables and berries per day in average proportions had a calculated HI of 0.15, 0.021 and 0.0003, under the assumption of imported conventional, domestic conventional, and organic products, respectively [78]. This indicates an at least 70 times lower exposure weighted by toxicity for a diet based on organic foods. There are several routes by which pesticides not approved for use in organic agriculture may contaminate organic products, including spray drift or volatilisation from neighbouring fields, fraudulent use, contamination during transport and storage in vessels or storages where previously conventional products have been contained, and mislabelling by intention or mistake. Overall, however, current systems for the certification and control of organic products ensure a low level of pesticide contamination as indicated by chronic and acute risks above, although they still can be improved [79].
The general populations exposure to several pesticides can be measured by analysing blood and urine samples, as is routinely done in the US [80] although not yet in Europe. However, a few scattered European studies from France [8183], Germany [84], the Netherlands [85], Spain [86], Belgium [87], Poland [88] and Denmark [89] have shown that EU citizens are commonly exposed to organophosphate and pyrethroid insecticides. A general observation has been higher urinary concentrations of pesticide metabolites in children compared to adults, most likely reflecting childrens higher food intake in relation to body weight and maybe also more exposure-prone behaviours. The urinary concentrations of generic metabolites of organophosphates (dialkyl phosphates, DAPs) and pyrethroids (3-phenoxybenzoic acid, 3-PBA) found in most of the European studies were similar to or higher than in the US studies. Although urinary metabolite concentration might overestimate the exposure to the parent compounds, due to ingestion of preformed metabolites in food items, several studies have reported associations between urinary metabolite concentrations and neurobehavioral deficits as described below. Besides, the metabolites are not always less toxic than the parent compounds [90].
For the general population, pesticide residues in food constitute the main source of exposure for the general population. This has been illustrated in intervention studies where the urinary excretion of pesticides was markedly reduced after 1week of limiting consumption to organic food [9193]. Similar conclusions emerged from studies investigating associations between urinary concentrations of pesticides and questionnaire information on food intake, frequency of different foodstuffs and organic food choices. Thus a high intake of fruit and vegetables is positively correlated with pesticide excretion [94], and frequent consumption of organic produce is associated with lower urinary pesticide concentration [95].
Pesticide exposure and health effects
The regulatory risk assessment of pesticides currently practised in the EU is comprehensive, as a large number of toxicological effects are addressed in animal and other experimental studies. Nonetheless, there are concerns that this risk assessment is inadequate at addressing mixed exposures, specifically for carcinogenic effects [96] as well as endocrine-disrupting effects [97, 98] and neurotoxicity [99]. Furthermore, there are concerns that test protocols lag behind independent science [100], studies from independent science are not fully considered [101] and data gaps are accepted too readily [102]. These concerns primarily relate to effects of chronic exposure and to chronic effects of acute exposure, which are generally more difficult to discover than acute effects. Most studies rely on urinary excretion of pesticide metabolites and a common assumption is that the subjects were exposed to the parent chemicals, rather than the metabolites.
The overall health benefits of high fruit and vegetable consumption are well documented [31, 35]. However,as recently indicated for effects on semen quality [103], these benefits might be compromised by the adverse effects of pesticide residues. When benefits are offset by a contaminant, a situation of inverse confounding occurs, which may be very difficult to adjust for [104]. The potential negative effects of dietary pesticide residues on consumer health should of course not be used as an argument for reducing fruit and vegetable consumption. Neither should nutrient contents be used to justify exposures to pesticides. Exposures related to the production of conventional crops (i.e. occupational or drift exposure from spraying) have been related to an increased risk of some diseases including Parkinsons disease [105107], type 2 diabetes [108, 109] and certain types of cancers including non-Hodgkin lymphoma [110] and childhood leukaemia or lymphomas, e.g. after occupational exposure during pregnancy [105, 111] or residential use of pesticides during pregnancy [105, 112] or childhood [113]. To which extent these findings also relate to exposures from pesticide residues in food is unclear. However, foetal life and early childhood are especially vulnerable periods for exposure to neurotoxicants and endocrine disruptors. Even brief occupational exposure during the first weeks of pregnancy, before women know they are pregnant, have been related to adverse long-lasting effects on their childrens growth, brain functions and sexual development, in a Danish study on greenhouse workers children [114118].
In order to assess the potential health risk for consumers associated with exposure to dietary pesticides, reliance onepidemiological studies of sensitive health outcomes and their links to exposure measuresis needed. Such studies are complicated both by difficult exposure assessment and the necessary long-term follow-up. The main focus so far has been on cognitive deficits in children in relation to their mothers exposure level to organophosphate insecticides during pregnancy. This line of research is highly appropriate given the known neurotoxicity of many pesticides in laboratory animal models [99] and the substantial vulnerability of the human brain during early development [119].
Most of the human studies have been carried out in the US and have focused on assessing brain functions in children in relation to prenatal organophosphate exposure. In a longitudinal birth cohort study among farmworkers in California (the CHAMACOS cohort), maternal urinary concentrations of organophosphate metabolites in pregnancy were associated with abnormal reflexes in neonates [120], adverse mental development at 2years of age [121], attention problems at three and a half and 5years [122], and poorer intellectual development at 7years [123]. In accordance with this, a birth cohort study from New York reported impaired cognitive development at ages 12 and 24months and 6 9years related to maternal urine concentrations of organophosphates in pregnancy [124]. In another New York inner-city birth cohort, the concentration of the organophosphate chlorpyrifos in umbilical cord blood was associated with delayed psychomotor and mental development in children in the first 7years of life [125], poorer working memory and full-scale IQ at 7years of age [126], structural changes, including decreased cortical thickness, in the brain of the children at school age [127], and mild to moderate tremor in the arms at 11years of age [128]. Based on these and similar studies, chlorpyrifos has recently been categorised as a human developmental neurotoxicant [129]. Recent reviews of neurodevelopmental effects of organophosphate insecticides in humans conclude that exposure during pregnancy at levels commonly found in the general population likely have negative effects on childrens neurodevelopment [130132]. In agreement with this conclusion, organophosphate pesticides considered to cause endocrine disruption contribute the largest annual health cost within the EU due to human exposures to such compounds, and these costs are primarily due to neurodevelopmental toxicity, as discussed below.
Since growth and functional development of the human brain continues during childhood, the postnatal period is also assumed to bevulnerable to neurotoxic exposures [119]. Accordingly, five-year-old children from the CHAMACOS cohort had higher risk scores for development of attention deficit hyperactive disorder (ADHD) if their urine concentration of organophosphate metabolites was elevated [122]. Based on cross-sectional data from the NHANES data base, the risk of developing ADHD increases by 55% for a ten-fold increase in the urinary concentration of organophosphate metabolites in children aged 8 to 15years [133]. Also based on the NHANES data, children with detectable concentrations of pyrethroids in their urine are twice as likely to have ADHD compared with those below the detection limit [134]. In addition, associations between urinary concentrations of pyrethroid metabolites in children and parent-reported learning disabilities, ADHD or other behavioural problems in the children have recently been reported in studies from the US and Canada [135, 136].
So far only few prospective studies from the EU addressing associations between urinary levels of pesticides and neurodevelopment in children from the general population have been published. Three studies are based on the PELAGIE cohort in France and present results for organophosphates and pyrethroids respectively [81, 82, 137]. While no adverse effects on cognitive function in six-year-old children were related to maternal urine concentrations of organophosphates during pregnancy, the concentration of pyrethroid metabolites was associated with internalising difficulties in the children at 6years of age. Also, the childrens own urinary concentrations of pyrethroid metabolites were related to decrements in verbal and memory functions and externalising difficulties and abnormal social behaviour. While this sole European study did not corroborateUSbirth cohort studies resultsshowing that exposure during pregnancy to organophosphate insecticides at levels found in the general population may harm brain development in the foetus, the exposure levels measured in the PELAGIE cohort were considerably lower for both organophosphates and pyrethroids than those measured in other European studies as well as in studies from the US and Canada. For example, the median urine concentration of organophosphate metabolites in pregnant women in the PELAGIE cohort was 2 6 times lower than for pregnant women in other studies [85, 122, 138] and the concentration of the common pyrethroid metabolite 3-PBA was only detectable in urine samples from 30% of the women compared to 8090% in other studies [88, 139]. Thus, to supplement the French study and the previously mentioned Danish study of greenhouse workers children, additional studies that include more representative exposure levels for EU citizens are desirable.
Although exposure levels found in European countries are generally similar to or slightly higher than concentrations found in the US studies, the risk of adverse effects on neurodevelopment in European populations needs to be further characterised. The organophosphate insecticides contributing to the exposure might differ between the US and the EU, also in regard to oral and respiratory intakes. According to the European Food Safety Agency (EFSA), of all the organophosphate insecticides,chlorpyrifos most often exceeds the toxicological reference value (ARfD) [74]. A recent report utilised US data on adverse effects on childrens IQ levels at school age to calculate the approximate costs of organophosphate exposure in the EU. The total number of IQ points lost due to these pesticides was estimated to be 13 million per year, representing a value of about 125 billion [140], i.e. about 1% of the EUs gross domestic product. Although there is some uncertainty associated with this calculation, it most likely represents an underestimation, as it focused only on one group of pesticides.
Unfortunately, epidemiological evidence linking pesticide exposure and human health effects is rarely regarded as sufficiently reliable to take into account in the risk assessment conducted by regulatory agencies. For example, the conclusion from the epidemiological studies on chlorpyrifos is that an association of prenatal chlorpyrifos exposure and adverse neurodevelopmental outcomes is likely, but that other neurotoxic agents cannot be ruled out, and that animal studies show adverse effects only at 1000-fold higher exposures [141]. A recent decrease of the maximum residue limit for chlorpyrifos in several crops [142, 143] was based on animal studies only [144], but the limits for the sister compound, chlorpyrifos-methyl were unchanged. This case highlights a major limitation to current approaches to protecting the general population against a broad variety of pesticides.
Production system and composition of plant foods
Fertilisation in organic agriculture is based on organic fertilisers such as farmyard manure, compost and green fertilisers, while some inorganic mineral fertilisers are used as supplements. Nitrogen (N) input is limited to 170kg/ha * year [5, 145]. In conventional agriculture, fertilisation is dominated by mineral fertiliser, although farmyard manure is also common in some countries. There is no general limit on N input. Typically, crop yield is limited by plant N availability in organic but not in conventional systems [146] Phosphorus (P) input is on average similar or slightly lower in organic systems [147].
In the absence of particular nutrient deficiency, focusing on single nutrients may be of limited value for evaluating the impact of a food or diet on human health [49]; studies of actual health effects, as discussed above, are generally more informative than studies of single nutrients.
Overall crop composition
Metabolomics [148152], proteomics [153, 154] and transcriptomics [155, 156] studies in controlled field trials provide evidence that the production system has an overall influence on crop development, although there is no direct relevance of these studies for human health. Furthermore, the generally lower crop yield in organic systems [146] as such indicates an effect of management strategy on plant development.
Several systematic reviews and meta-analyses [25, 157159] with different scopes, inclusion criteria and statistical methods have summarised several hundred original studies reporting some aspect of plant chemical composition in relation to conventional and organic production, in search of overall trends across crops, varieties, soils, climates, production years etc. While the overall conclusions of these systematic reviews look contradictory at first sight, there is agreement between them in most of the detailed findings:
Nitrogen and phosphorus
Existing systematic reviews have consistently found lower total nitrogen (7% [157], 10% [159]) and higher phosphorus (standardised mean difference (SMD) 0.82 [25], 8% [157]) in organic compared to conventional crops. These findings lack direct relevance for human health. However, considering the differences in fertilisation strategies discussed above, and the fundamental importance of N, P [160162], and the N:P ratio [163] for plant development, this may lend some plausibility to other observed effects of the production system on crop composition.
Vitamins
Systematic reviews generally agree that the concentration of macronutrients, vitamins, and minerals in crops is either not at all or only slightly affected by the production system. For example, ascorbic acid (vitamin C) has received most attention in this context. Meta-analyses report only small effect sizes of the organic production system on vitamin C content [25, 158, 159].
Polyphenols
(Poly)phenolic compounds are not essential nutrients for humans but may play a role in preventing several non-communicable diseases, including cardiovascular disease, neurodegeneration and cancer [164]. The detailed mechanisms are complex and not fully understood [164]. Several environmental and agronomic practices affect the phenolic composition of the crop, including light, temperature, availability of plant nutrients and water management [165]. Under conditions of high nitrogen availability, many plant tissues show a decreased content of phenolic compounds, although there are examples of an opposite relationship [165].
Meta-analyses report modest effect sizes of the production system on total phenolics content, e.g. an increase of 14 26% [25, 158, 159]. For some narrower groups of phenolic compounds, larger relative concentration differences (in percent) between organic and conventional crops have been reported [159]. However, such findings represent unweighted averages typically from small and few studies, and are therefore less reliable.
Collectively the published meta-analyses indicate a modestly higher content of phenolic compounds in organic food, but the evidence available does not constitute a sufficient basis for drawing conclusions on positive effects of organic compared to conventional plant products in regard to human health.
Cadmiumand other toxic metals
Cadmium (Cd) is toxic to the kidneys, can demineralise bones and is carcinogenic [166]. Cd is present naturally in soils, and is also added to soils by P fertilisers and atmospheric deposition. Several factors, including soil structure and soil chemistry, humus content and pH, affect the plant availability of Cd [167]. The application of Cd-containing fertilisers increases Cd concentrations in the crops [167, 168]. Low soil organic matter generally increases the availability of Cd for crops [169], and organically managed farms tend to have higher soil organic matter than conventionally managed farms [11].
The source of Cd in mineral fertilisers is the raw material phosphate rock. The European average Cd content in mineral fertilisers is reported as 68mg Cd/kg P [170] or 83mg Cd/kg P [171]. The content of Cd in farmyard manure is variable but apparently in many cases lower: Various types of animal manure in a German collection averaged between 14 and 37mg Cd/kg P [172].
Smith-Spangler et al. [25] found no significant difference in the Cd content of organic and conventional crops (SMD=0.14, 95% CI -0.74 0.46) in their meta-analysis, while Baraski et al. [159] report significantly 48% higher Cd concentration in conventional compared to organic crops (SMD=-1.45, 95% CI -2.52 to 0.39) in another meta-analysis largely based on the same underlying original studies, albeit with different inclusion criteria. We contacted the authors of these meta-analyses in order to understand this discrepancy. An updated version of the Baraski meta-analysis, in which some inconsistencies have been addressed and which has been provided by the original authors [173], shows a significant 30% (SMD=0.56, 95% CI -1.08 to 0.04) elevations of Cd contents in conventional compared to organic crops; in subgroup analysis, this difference is restricted to cereal crops. No updated meta-analysis was available for Smith-Spanglers analysis [25]; apparently, two large well-designed studies with tendencies towards a lower Cd content in organic crops were not considered [174, 175] although they appear to fulfil the inclusion criteria. Also, a correction for multiple testing has been imposed, which may be overly conservative, given the prior knowledge that mineral fertilisers constitute an important source of Cd to soils and crops. It is unclear how these points would affect the results of Smith-Spanglers meta-analysis.
There are short-term and long-term effects of Cd influx from fertilisers on the Cd content of crops [167] but no long-term study comparing Cd content in organic and conventional crops is available. In absence of such direct evidence, two long-term experiments indicate a higher slope in Cd concentration over time for minerally fertilised compared to organically fertilised cereal crops [176, 177], after over 100years of growing.
A lower Cd content of organic crops is therefore plausible due to a lower Cd content in the fertilisers used in organic farming, and potentially due to higher soil organic matter in organic farmland. The general populations Cd exposure is close to, and in some cases above, the tolerable intake and therefore their exposure to Cd should be reduced. For non-smokers, food is the primary source of exposure, with cereals and vegetables being the most important contributors [168].
For other toxic metals including lead, mercury and arsenic, no differences in concentration in organic and conventional crops have been reported [25, 159]. Uranium (U) is also present as a contaminant of concern in mineral P fertilisers [178], but less so in organic fertilisers [179], and consequently manure-based cropping systems have a lower U load than mineral-fertilised systems at equal P load [179]. Uranium appears to accumulate in mineral-fertilised soils [180], and agricultural activity may increase the U content of surface and groundwater [181, 182]. However, no evidence was found comparing uranium contents of organic and conventional products.
Fungal toxins
Regarding fungal toxins in crops, one meta-analysis has reported a lower contamination of organic compared to conventional cereal crops with deoxynivalenol (DON), produced by certain fusarium species [25]. Although not fully understood, fungicide applications may alter fungal communities on cereal leaves, potentially weakening disease-suppressive species [183, 184]. Also, crop rotations including non-cereal crops may contribute to lower infestation with fusarium [185], while N availability is positively associated with cereal DON content [186]. These factors give plausibility to the observed lower DON contamination in organic cereals. In the EU, the mean chronic exposure of toddlers, infants and children to DON is above the tolerable daily intake (TDI), with grains and grain-based products being the main contributors to total exposure. The TDI is based on decreased body weight gain observed in mice [187]. The production system does not have any observed effect on the concentration of ochratoxin A (OTA), another fungal toxin of importance in cereal production [25].
Animal-based foods
By regulation, herbivores in organic production receive at least 60% of their feed intake as roughage on a dry matter basis. Depending on the seasonal availability of pastures, roughage can be fresh, dried, or silage. Also omnivores in organic production receive roughage as part of their daily feed, and poultry has access to pasture [6]. Corresponding regulations are for the most part missing in conventional animal production. In consequence, feeding strategies in organic animal production include a higher fraction of roughage compared to conventional systems, e.g. for dairy cows [188, 189].
Fatty acids
Much of the focus of existing research on compositional differences of organic and conventional animal-based foods is on the fatty acid composition, with a major interest in omega-3 FAs due to their importance for human health. Some studies also address the content of minerals and vitamins.
The FA composition of the feed is a strong determinant of the fatty acid composition of the milk, egg or meat [190, 191]. Grass and red clover, typical roughage feeds, contain between 30% and 50% omega-3 FA of total FA, while the concentrate feeds cereals, soy, corn, and palm kernel cake all contain below 10% omega-3 FA of total FA [190]. Like humans, farm animals turn a small part of dietary alpha-linolenic acid into long-chain omega-3 fatty acids with the help of elongase and desaturase enzymes.
For cows milk, a recent meta-analysis reports conclusively an approximately 50% higher content of total omega-3 fatty acids (as percent of total fatty acids) in organic compared to conventional milk [192], generally confirming earlier reviews [25, 189]. Also, the content of ruminant FAs (a group of natural trans FAs produced in the cows rumen) is higher in organic milk. The content of saturated fatty acids, mono-unsaturated fatty acids and omega-6 PUFA was similar in organic and conventional milk [192].
A considerable statistical heterogeneity in these findings is reported. Individual differences described above are based on results from between 11 and 19 included studies. The observed differences are plausible, because they are directly linked to differences in feeding regimens. It should also be noted that several other factors influence the fatty acid composition in milk [193]. Specifically, the season (indoor vs. outdoor) has an impact on the feeding regime [188] and therefore on the omega-3 content of milk. However, the content of omega-3 fatty acids is higher in organic milk during both the outdoor and indoor seasons [189].
For eggs, it is likewise well described that the FA composition of the feed [190] and consequently the access to pasture [194, 195] such as in organic systems, is a strong determinant of the fatty acid composition of the egg. However, only few studies have compared the FA composition in organic and conventional eggs [196] and a systematic review is not available. A higher omega-3 content of organic eggs is plausible but has not been documented.
A total of 67 original studies report compositional aspects of meat (mainly beef, chicken, lamb, and pork) from organic and conventional husbandry and were recently summarised in a meta-analysis [197]. Based on 23 and 21 studies respectively, the content of total PUFA and omega-3 PUFA was found to be significantly higher (23 and 47%, respectively) in organic compared to conventional meats. Weighted by average consumption in Europe, choosing organic instead of conventional meat, while maintaining a constant consumption, increased the intake of PUFA and omega-3 FA from meat by 17 and 22%, respectively [198]. These findings are plausible, especially in the case of omega-3 PUFA, considering the known differences in feeding regimens in organic and conventional production. However, few studies were available for each analysis, leaving many analyses with high uncertainty and poor statistical power. Furthermore, fatty acid metabolism differs between ruminants and monogastric animals [190]. Also, the actual differences in feeding regimens between conventionally and organically raised animals may differ by species, and by country. The variation between studies and between species was large, and the overall reliability of these results is therefore lower compared to milk above. This meta-analysis therefore indicates a plausible increase in omega-3 contents in organic meats, but more well-designed studies are needed to confirm this effect [197].
Dairy products account for 45% of the total PUFA intake in most European populations, while meat and meat products contribute another 723% [199]. The contribution of milk fat to omega-3 PUFA intake (approximated as intake of -linolenic acid) has been estimated at 516% [200, 201], while meat contributes with 1217% [201, 202]. The effect of exchanging organic for conventional dairy products on omega-3 PUFA intake while maintaining a constant consumption has not been examined rigorously. From the intake and composition data presented here, it can be estimated that choosing organic products would increase the average dietary omega-3 PUFA intake by 2.58% (dairy) and by a less certain 2.54% (meat). A recent preliminary estimate based on FAO food supply data resulted in similar numbers [198]. For certain population groups and fatty acids, these numbers could be higher, and an increased omega-3 PUFA consumption is generally desirable, as some subpopulations have a lower-than-recommended intake of omega-3 PUFA [203]. However, overall, the effect of the animal production system on omega-3 PUFA intake is minor, and no specific health benefits can be derived. Furthermore, other dietary omega-3 PUFA sources, specifically certain plant oils and fish, are available that carry additional benefits [204206]. The existence of specific health benefits of ruminant trans fatty acids (as opposed to industrial trans fatty acids) is indicated by some studies [207] but not strongly supported [208]. Taking into account the actually consumed amountsof ruminant trans fatty acids, this is likely lacking public health relevance [208].
Trace elements and vitamins
A recent meta-analysis points to a significantly higher content of iodine (74%) and selenium (21%) in conventional milk and of iron (20%) and tocopherol (13%) in organic milk based on six, four, eight and nine studies respectively [192]. Iodine deficiency during pregnancy and infancy leads to impairment of brain development in the offspring, while excess iodine intake is associated with similar effects, and the window of optimal iodine intake is relatively narrow [209]. Overall, iodine intake in Europe is low and mild deficiency is prevalent [210]. The preferred way of correcting deficiency is salt iodisation [210, 211], because salt is consumed almost universally and with little seasonal variation [212].
Feed iodine supplementation is not linked by regulation to the production system in the EU, as iodine is listed as approved feed additive, and the maximum amount of supplementation is the same for all milk production. Optimum dairy cow supplementation should be seen in relation to other national strategies for human iodine intake. This should also take into account human subpopulations with low or no intake of dairy products.
For tocopherol, selenium and iron, a higher content is generally desirable, and in the case of selenium milk is an important source. However, the concentration differences between organic and conventional milk are modest and based on a few studies only.
Antibiotic resistant bacteria
Overly prevalent prophylactic use of antibiotics in animal production is an important factor contributing to increasing human health problems due to resistant bacteria. Antibiotic use is strongly restricted in organic husbandry, which instead aims to provide good animal welfare and enough space in order to promote good animal health.
Antibiotics constitute an integral part of intensive animal production today, and farm animals may act as important reservoirs of resistant genes in bacteria [213, 214]. It is reported that a substantial proportion (50 80%) of antibiotics are used for livestock production worldwide [215]. On a per kg biomass basis, in 2014, the amount of antimicrobial drugs consumed by farm animals was slightly higher than the antimicrobial drugs used for humans in the 28 EU/EEA countries surveyed, with substantial differences between countries regarding volumes and types of substances [216].
In recent decades, there have been increasing concerns that the use of antibiotics in livestock would contribute to impairing the efficiency of antibiotic treatment in human medical care [217]. Despite the lack of detailed information on transmission routes for the vast flora of antibiotic-resistant bacteria and resistance genes, there is a global need for action to reduce the emerging challenges associated with the reduced efficiency of antibiotics and its consequences for public health, as well as for the environment more generally [218, 219].
The use of antibiotics may increase the economic outcome of animal production [220, 221], but the spreading of multi-resistant genes is not just a problem for the animal production sector alone. Negative effects are affecting parts of society not directly associated with livestock production. This means that the costs of side effects are borne by society in general and not primarily by the agricultural sector. However, the generalisation cannot be made that all antibiotic treatment in farm animals represents a hazard to public health [222, 223].
The use of antibiotics in intensive livestock production is closely linked to the housing and rearing conditions of farm animals. Specific conditions for conventional livestock farming in different countries, as well as farmers attitudes, may differ between countries, e.g. conventional pig production at above EU animal welfare standards and farmers attitudes in Sweden [224, 225]. Conventional production is typically aiming for high production levels with restricted input resources such as space, feed etc., and these conditions may cause stress in the individual animal as it is unable to cope with the situation, e.g. in pig production [226, 227]. This means that higher stocking density, restricted space and barren environment are factors increasing the risk of the development of diseases, and therefore it is more likely that animals under these conditions need antibiotic treatments.
Organic production aims for less intensive animal production, which generally means that the animals have access to a more spacious and enriched environment, access to an outdoor range and restricted group sizes, and other preconditions [70]. This would ultimately decrease the need for preventive medication of the animals as they can perform more natural behaviours and have more opportunity to maintain a good health. However, in practice, the health status of organic livestock is complex and disease prevention needs to be adapted to the individual farm [228]. A report on the consequences of organic production in Denmark demonstrates that meeting the requirements of organic production has several positive consequences in relation to animal welfare and health [70].
According to EU regulations, routine prophylactic medication of animals in organic production is not allowed. However, diseases should be treated immediately to avoid suffering, and the therapeutic use of antibiotics is allowed, but with longer withdrawal periods than in conventional production [5]. Furthermore, products from animals treated more than three times during 12months, or, if their productive lifecycle is less than 1year, more than once, cannot be sold as organic [6]. This means that therapeutically the same antibiotics used in conventional farming may be used in organic farming, but under different conditions. For example, antibiotics mainly used for sub-therapeutic treatment as prophylaxis are never considered in organic production.
While the organic regulations aim for a low use of antibiotics in livestock production, the actual use of antibiotic drugs in European organic compared to conventional animal husbandry is not comprehensively documented. Scattered studies indicate that the antibiotic use generally is substantially higher in conventional compared to organic systems, especially for pigs (approximately 5 15-fold higher) [229, 230]. In studies from Denmark [231] and the Netherlands [232], the antibiotic use in dairy cows was 50% and 300% higher in conventional compared to organic systems, although a Swedish study found no differences in disease treatment strategies between organic and conventional dairy farms, e.g. for mastitis [233]. While only sparingly documented (e.g. [234, 235]), there is only little use of antibiotics in EU organic broiler production. This is a consequence of regulations prohibiting prophylactic use and prescribing long withdrawal periods before slaughter [6, 236], in conjunction with the fact that it is not feasible to treat single animals in broiler flocks. In conventional broiler production, antibiotic use is common (e.g. [237239]).
Recently, gene sequencing has revealed that the routes of transmission of resistance genes between human and farm animal reservoirs seem to be complex [213, 222, 240]. Nevertheless, a recent EFSA report found that in both humans and animals, positive associations between consumption of antimicrobials and the corresponding resistance in bacteria were observed for most of the combinations investigated [241], which has subsequently been strengthened [216]. In addition to direct transmission between animals and humans via contact or via food, resistant strains and resistance genes may also spread into the environment [242].
Previously, it has been postulated that a reduced need and use of antibiotics in organic livestock production will diminish the risk of development of antibiotic resistance [243], and this has also been demonstrated with regard to resistant E. coli in organic pigs compared to conventional pigs [244]. It has also been shown that the withdrawal of prophylactic use of antibiotics when poultry farms are converted from conventional to organic production standards leads to a decrease in the prevalence of antibiotic-resistant Salmonella [245].
Resistant bacteria may be transferred within the production chain from farm to fork [246]. It has been found that organic livestock products are less likely to harbour resistant bacteria in pork and chicken meat [25].
In pig production, particular attention has been paid to methicillin-resistant Staphylococcus aureus (MRSA), and in Dutch and German studies, for example, MRSA has been isolated in 30 and 55% respectively of all pigs tested [247, 248]. Furthermore, it has been found that healthy French pig farmers are more likely to carry MRSA than control persons [249] and that they carry similar strains of MRSA to those found on their pig farms [250]. However, the prevalence of MRSA in pig production may differ between conventional and organic farms, and in a meta-study in 400 German fattening pig herds, the odds ratio (OR) for MRSA prevalence was 0.15 (95% CI 0.04, 0.55) in organic (n=23) compared to conventional (n=373) pig farms [248]. Multivariate adjustment for potential risk factors rendered this association non-significant, suggesting that it was carried by other factors, including factors that are regulated in or associated with organic production, such as non-slatted floors, no use of antibiotics, and farrow-to-finish herd types. Furthermore, even if there are considerable differences in antibiotic use between countries, it has been found that antibiotic resistance is less common in organic pigs compared to conventional pigs in France, Italy, Denmark, and Sweden [251, 252].
Although it is rare for conventional farms to adopt knowledge about management and housing from organic production except when converting farms in line with organic standards, there may be options to improve animal health and welfare by knowledge transfer to conventional farms in order to reduce the use of antibiotics [253].
Within organic production, labelling requires full traceability in all steps in order to guarantee the origin of the organic products being marketed [5]. Application of the general principle of organic regulations about transparency throughout the food chain can be used to mitigate emerging problems of transmission of antimicrobial resistance. However, transition to organic production for the whole livestock sector would, on its own, be only part of a solution to the antibiotics resistance issue, because factors outside animal production, such as their use in humans, will be unaffected.
Discussion
An assessment of the human health effects associated with diets based on organic food production must rely on two sets of evidence. The first set of evidence is the epidemiological studies comparing population groups with dietary habits that differ substantially in regard to choices of organic v. conventional products. These studies are to some extent complemented by experimental studies using animal models and in vitro models. The second set of data relies on indirect evidence such as chemical analyses of food products and their contents of nutrients and contaminants or antibiotic use and resistance patterns, in onsequence of agricultural production methods. Both sets of results are associated with certain strengths and weaknesses.
The few human studies that have directly investigated the effects of organic food on human health have so far yielded some observations, including indications of a lower risk of childhood allergies, adult overweight/obesity [18, 46] and non-Hodgkin lymphoma (but not for total cancer) [37] in consumers of organic food. Owing to the scarcity or lack of prospective studies and the lack of mechanistic evidence, it is presently not possible to determine whether organic food plays a causal role in these observations. However, it has also been observed that consumers who prefer organic food have healthier dietary patterns overall, including a higher consumption of fruit, vegetables, whole grains, and legumes and a lower consumption of meat [18, 29, 37]. This leads to some methodological difficulties in separating the potential effect of organic food preference from the potential effect of other associated lifestyle factors, due to residual confounding or unmeasured confounders. These dietary patterns have in other contexts been associated with a decreased risk of several chronic diseases, including diabetes and cardiovascular disease [3036]. It is therefore expected that consumers who regularly eat organic food have a decreased risk of these diseases compared to people consuming conventionally-produced food, as a consequence of dietary patterns. These dietary patterns appear also to be more environmentally sustainable than average diets [254].
Food analyses tend to support the notion that organic foods may have some health benefits. Consumers of organic food have a comparatively low dietary exposure to pesticides. Although chemical pesticides undergo a comprehensive risk assessment before market release in the EU, there are important gaps in this risk assessment. In some cases, specifically for cognitive development during childhood as an effect of organophosphate insecticide exposure during pregnancy, epidemiological studies provide evidence of adverse effects [140, 255]. Organic agriculture allows for lower pesticide residues infood and may be instrumental in conventional agricultures transition towards integrated pest management by providing a large-scale laboratory for non-chemical plant protection.
This review emphasizes that pesticide exposure from conventional food production constitutes a main health concern. A key issue that has only recently been explored in biomedical research is that early-life exposure is of major concern, especially prenatal exposure that may harm brain development. Most insecticides are designed to be toxic to the insect nervous system, but many higher species depend on similar neurochemical processes and may therefore all be vulnerable to these substances [129]. Besides insecticides, experimental studies suggest a potential for adverse effects on the nervous system for many herbicides and fungicides as well [99]. However, no systematic testing is available since testing for neurotoxicity especially developmental neurotoxicity has not consistently been required as part of the registration process, and allowable exposures may therefore not protect against such effects. At least 100 different pesticides are known to cause adverse neurological effects in adults [129], and all of these substances must therefore be suspected of being capable of damaging also developing brains. The need for prevention of these adverse outcomes is illustrated by the recent cost calculations [140] and the additional risk that pesticide exposures may lead to important diseases, such as Parkinsons disease, diabetes and certain types of cancer.
The outcomes in children and adults and the dose-dependences are still incompletely documented, but an additional limitation is the lack of exposure assessments in different populations and also their association with dietary habits. The costs from pesticide use in regard to human health and associated costs to society are likely to be greatly underestimated due to hidden and external costs, as recently reviewed [256]. Also, gaps in the regulatory approval process of pesticides may lead to important effects being disregarded and remaining undetected.
In regard to nutrients, organic dairy products, and probably also meat, have an approximately 50% higher content of omega-3 fatty acids compared to conventional products. However, as these products only are a minor source of omega-3 fatty acids in the average diet, the nutritional significance of this effect is probably low (although this has not been proven). The nutritional content of crops is largely unaffected by the production system, according to current knowledge. Vitamins and minerals are found in similar concentrations in crops from both systems. One exception is the increased content of phenolic compounds found in organic crops, although this is still subject to uncertainty despite a large number of studies that have addressed this issue. Accordingly, although in general being favourable for organic products, the established nutritional differences between organic and conventional foods are small, and strong conclusions for human health cannot currently be drawn from these differences. There are indications that organic crops contain less cadmium compared to conventional crops. This is plausible, primarily because mineral fertiliser is an important source of cadmium in soils. However, notably, long-term farm pairing studies or field trials that are required for definitely establishing or disproving this relationship are lacking. Owing to the high relevance of cadmium in food for human health, this lack of research constitutes an important knowledge gap.
With respect to the development of antibiotic resistance in bacteria, organic animal production may offer a way of restricting the risks posed by intensive production, and even decreasing the prevalence of antibiotic resistance. Organic farm animals are less likely to develop certain diseases related to intensive production compared to animals on conventional farms. As a consequence, less antibiotics for treating clinical diseases are required under organic management, where their prophylactic use also is strongly restricted. This decreases the risk for development of antibiotic resistance in bacteria. Furthermore, the transparency in organic production may be useful for acquiring knowledge and methods to combat the rising issues around transmission of antimicrobial resistance within food production.
It appears essential that use of antibiotics in animal production decreases strongly or completely ceases in order to decrease the risk of entering a post-antibiotic era. The development and upscaling of rearing systems free or low in antibiotic use, such as organic broiler production, may be an important contribution of organic agriculture to a future sustainable food system.
Most of the studies considered in this review have investigated the effects of agricultural production on product composition or health. Far less attention has been paid to the potential effects of food processing. Processing may affect the composition of foods and the bioavailability of food constituents. It is regulated [5] and recognised [257] that food additives are restricted for organic products compared to conventional products. It is also recognised that the degree of food processing may be of relevance to human health [258, 259]. In organic food processing, the processing should be done with care, preferably with the use of biological, mechanical and physical methods [5] but there are no specific restrictions or guidelines. With the exception of chemical additives, it is unknown whether certain food processing methods (e.g. fermentation of vegetables, pasteurisation of vegetables) are more prevalent in organic or conventional products or consumption patterns, or whether such differences are of relevance to human health.
The scopes of two recent reports, from Norway [260] and Denmark [70], in part overlap with the present work. Broadly, the reviewed results and conclusions presented in those reports are in line with this article. For several topics, important new evidence has been published in recent years. Consequently, in some cases stronger conclusions can be drawn today. Furthermore, the present review includes epidemiological studies of pesticide effects in the evidence base reviewed.
Over all, the evidence available suggested some clear and some potential advantages associated with organic foods. The advantages in general do not necessarily require organic food production as strictly defined in current legislation. Certain production methods, such as changes in the use of pesticides and antibiotics, can be implemented in conventional production, e.g. supporting a development towards a sustainable use of pesticides [261]. Thereby, practices and developments in organic agriculture can have substantial public health benefits also outside the organic sector.
Diet choices and the associated food production methods also have important impacts on environmental sustainability [254]. Consumption patterns of consumers preferring organic food [16, 18, 19, 37, 47] seem to align well with sustainable diets [2]. These consumption patterns also show some similarities with the Mediterranean Diet [262265] and with the New Nordic Diet [266269], with lower dietary footprints in regard to land use, energy and water consumption, and greenhouse gas emissions compared to concurrent average diets. Further evaluation is needed to assess the extent to which organic food systems can serve as example of a sustainable food systems [270].
For the development of healthy and environmentally-sustainable food systems in thefuture, production and consumption need to be considered in an integrated manner [2, 271]. While an evaluation of overall impacts of different food systems on environmental sustainability would be highly desirable [270], the present review has attempted to assess the human health issues in regard to organic production methods and consumer preferences for organic food, both important aspects of sustainability.
References
2. Burlingame B, Dernini S. Food and Agriculture Organization of the United Nations (FAO): Sustainable diets and biodiversity. Directions and solutions for policy, research and action. In. Edited by Burlingame B, Dernini S; 2012.
4. The World of Organic Agriculture. Statistics and emerging trends. Frick and Bonn: FiBL and IFOAM organics international; 2017.
5. Council of the European Union: Council Regulation No 834/2007 of 28 June 2007 on organic production and labelling of organic products and repealing Regulation (EEC) No 2092/91. In: Off J Eur Union 2007.
6. European Commission: Commission Regulation (EC) No 889/2008 of 5 September 2008 laying down detailed rules for the implementation of Council Regulation (EC) No 834/2007 on organic production and labelling of organic products with regard to organic production, labelling and control. In: Off J Eur Union 2008.
7. Willer H, Schaack D, Lernoud J: Organic Farming and Market Development in Europe and the European Union. In: The World of Organic Agriculture - Statistics and Emerging Trends 2017. Edited by Willer H, Lernoud J. Frick and Bonn: FiBL and IFOAM; 2017.
11.
Reganold JP, Wachter JM. Organic agriculture in the twenty-first century. Nat Plants. 2016;2:15221. [PubMed] [Google Scholar]12.
Seufert V, Ramankutty N. Many shades of gray-the context-dependent performance of organic agriculture. Sci Adv. 2017;3(3):e1602638. [PMC free article] [PubMed] [Google Scholar]13.
Tscharntke T, Clough Y, Wanger TC, Jackson L, Motzke I, Perfecto I, Vandermeer J, Whitbread A. Global food security, biodiversity conservation and the future of agricultural intensification. Biol Conserv. 2012;151(1):5359. [Google Scholar]14.
Wheeler T, von Braun J. Climate change impacts on global food security. Science. 2013;341(6145):508513. [PubMed] [Google Scholar]15.
Oates L, Cohen M, Braun L. Characteristics and consumption patterns of Australian organic consumers. J Sci Food Agric. 2012;92(14):27822787. [PubMed] [Google Scholar]16.
Baudry J, Mejean C, Alles B, Peneau S, Touvier M, Hercberg S, Lairon D, Galan P, Kesse-Guyot E. Contribution of organic food to the diet in a large sample of French adults (the NutriNet-Sante cohort study) Nutrients. 2015;7(10):86158632. [PMC free article] [PubMed] [Google Scholar]17.
Baudry J, Touvier M, Alles B, Peneau S, Mejean C, Galan P, Hercberg S, Lairon D, Kesse-Guyot E. Typology of eaters based on conventional and organic food consumption: results from the NutriNet-Sante cohort study. Br J Nutr. 2016;116(4):700709. [PubMed] [Google Scholar]18.
Kesse-Guyot E, Peneau S, Mejean C, Szabo de Edelenyi F, Galan P, Hercberg S, Lairon D. Profiles of organic food consumers in a large sample of French adults: results from the Nutrinet-Sante cohort study. PLoS One. 2013;8(10):e76998. [PMC free article] [PubMed] [Google Scholar]19.
Eisinger-Watzl M, Wittig F, Heuer T, Hoffmann I. Customers purchasing organic food - do they live healthier? Results of the German National Nutrition Survey II. Eur J Nutr Food Saf. 2015;5(1):5971. [Google Scholar]20.
Hughner RS, McDonagh P, Prothero A, Shultz CJ, Stanton J. Who are organic food consumers? A compilation and review of why people purchase organic food. J Consum Behav. 2007;6(23):94110. [Google Scholar]21.
van de Vijver LP, van Vliet ME. Health effects of an organic diet--consumer experiences in the Netherlands. J Sci Food Agric. 2012;92(14):29232927. [PubMed] [Google Scholar]22.
Brown E, Dury S, Holdsworth M. Motivations of consumers that use local, organic fruit and vegetable box schemes in Central England and southern France. Appetite. 2009;53(2):183188. [PubMed] [Google Scholar]23.
Arvola A, Vassallo M, Dean M, Lampila P, Saba A, Lahteenmaki L, Shepherd R. Predicting intentions to purchase organic food: the role of affective and moral attitudes in the theory of planned behaviour. Appetite. 2008;50(23):443454. [PubMed] [Google Scholar]24.
Dangour AD, Lock K, Hayter A, Aikenhead A, Allen E, Uauy R. Nutrition-related health effects of organic foods: a systematic review. Am J Clin Nutr. 2010;92(1):203210. [PubMed] [Google Scholar]25.
Smith-Spangler C, Brandeau ML, Hunter GE, Bavinger JC, Pearson M, Eschbach PJ, Sundaram V, Liu H, Schirmer P, Stave C, et al. Are organic foods safer or healthier than conventional alternatives?: a systematic review. Ann Intern Med. 2012;157(5):348366. [PubMed] [Google Scholar]26.
Forman J, Silverstein J. Organic foods: health and environmental advantages and disadvantages. Pediatrics. 2012;130(5):e1406e1415. [PubMed] [Google Scholar]27.
Mark AB, Poulsen MW, Andersen S, Andersen JM, Bak MJ, Ritz C, Holst JJ, Nielsen J, de Courten B, Dragsted LO, et al. Consumption of a diet low in advanced glycation end products for 4 weeks improves insulin sensitivity in overweight women. Diabetes Care. 2014;37(1):8895. [PubMed] [Google Scholar]28.
Soltoft M, Bysted A, Madsen KH, Mark AB, Bugel SG, Nielsen J, Knuthsen P. Effects of organic and conventional growth systems on the content of carotenoids in carrot roots, and on intake and plasma status of carotenoids in humans. J Sci Food Agric. 2011;91(4):767775. [PubMed] [Google Scholar]29.
Torjusen H, Brantsaeter AL, Haugen M, Alexander J, Bakketeig LS, Lieblein G, Stigum H, Naes T, Swartz J, Holmboe-Ottesen G, et al. Reduced risk of pre-eclampsia with organic vegetable consumption: results from the prospective Norwegian mother and child cohort study. BMJ Open. 2014;4(9):e006143. [PMC free article] [PubMed] [Google Scholar]30.
Abete I, Romaguera D, Vieira AR, Lopez de Munain A, Norat T. Association between total, processed, red and white meat consumption and all-cause, CVD and IHD mortality: a meta-analysis of cohort studies. Br J Nutr. 2014;112(5):762775. [PubMed] [Google Scholar]31.
Boeing H, Bechthold A, Bub A, Ellinger S, Haller D, Kroke A, Leschik-Bonnet E, Muller MJ, Oberritter H, Schulze M, et al. Critical review: vegetables and fruit in the prevention of chronic diseases. Eur J Nutr. 2012;51(6):637663. [PMC free article] [PubMed] [Google Scholar]32.
Larsson SC, Orsini N. Red meat and processed meat consumption and all-cause mortality: a meta-analysis. Am J Epidemiol. 2014;179(3):282289. [PubMed] [Google Scholar]33.
Li F, Hou LN, Chen W, Chen PL, Lei CY, Wei Q, Tan WL, Zheng SB. Associations of dietary patterns with the risk of all-cause, CVD and stroke mortality: a meta-analysis of prospective cohort studies. Br J Nutr. 2015;113(1):1624. [PubMed] [Google Scholar]34.
Schwingshackl L, Hoffmann G. Diet quality as assessed by the healthy eating index, the alternate healthy eating index, the dietary approaches to stop hypertension score, and health outcomes: a systematic review and meta-analysis of cohort studies. J Acad Nutr Diet. 2015;115(5):780800.e785. [PubMed] [Google Scholar]35.
Wang X, Ouyang Y, Liu J, Zhu M, Zhao G, Bao W, Hu FB. Fruit and vegetable consumption and mortality from all causes, cardiovascular disease, and cancer: systematic review and dose-response meta-analysis of prospective cohort studies. BMJ. 2014;349:g4490. [PMC free article] [PubMed] [Google Scholar]36.
Zong G, Gao A, Hu FB, Sun Q. Whole grain intake and mortality from all causes, cardiovascular disease, and cancer: a meta-analysis of prospective cohort studies. Circulation. 2016;133(24):23702380. [PMC free article] [PubMed] [Google Scholar]37.
Bradbury KE, Balkwill A, Spencer EA, Roddam AW, Reeves GK, Green J, Key TJ, Beral V, Pirie K. The million women study C: organic food consumption and the incidence of cancer in a large prospective study of women in the United Kingdom. Br J Cancer. 2014;110(9):23212326. [PMC free article] [PubMed] [Google Scholar]38.
Alfven T, Braun-Fahrlander C, Brunekreef B, von Mutius E, Riedler J, Scheynius A, van Hage M, Wickman M, Benz MR, Budde J, et al. Allergic diseases and atopic sensitization in children related to farming and anthroposophic lifestyle--the PARSIFAL study. Allergy. 2006;61(4):414421. [PubMed] [Google Scholar]39.
Kummeling I, Thijs C, Huber M, van de Vijver LP, Snijders BE, Penders J, Stelma F, van Ree R, van den Brandt PA, Dagnelie PC. Consumption of organic foods and risk of atopic disease during the first 2 years of life in the Netherlands. Br J Nutr. 2008;99(3):598605. [PubMed] [Google Scholar]40.
Rist L, Mueller A, Barthel C, Snijders B, Jansen M, Simoes-Wust AP, Huber M, Kummeling I, von Mandach U, Steinhart H, et al. Influence of organic diet on the amount of conjugated linoleic acids in breast milk of lactating women in the Netherlands. Br J Nutr. 2007;97(4):735743. [PubMed] [Google Scholar]41.
Stenius F, Swartz J, Lilja G, Borres M, Bottai M, Pershagen G, Scheynius A, Alm J. Lifestyle factors and sensitization in children - the ALADDIN birth cohort. Allergy. 2011;66(10):13301338. [PubMed] [Google Scholar]42.
Fagerstedt S, Hesla HM, Ekhager E, Rosenlund H, Mie A, Benson L, Scheynius A, Alm J. Anthroposophic lifestyle is associated with a lower incidence of food allergen sensitization in early childhood. J Allergy Clin Immunol. 2016;137(4):12531256.e1251. [PubMed] [Google Scholar]43.
Alm JS, Swartz J, Lilja G, Scheynius A, Pershagen G. Atopy in children of families with an anthroposophic lifestyle. Lancet. 1999;353(9163):14851488. [PubMed] [Google Scholar]44.
Floistrup H, Swartz J, Bergstrom A, Alm JS, Scheynius A, van Hage M, Waser M, Braun-Fahrlander C, Schram-Bijkerk D, Huber M, et al. Allergic disease and sensitization in Steiner school children. J Allergy Clin Immunol. 2006;117(1):5966. [PubMed] [Google Scholar]45.
Thijs C, Muller A, Rist L, Kummeling I, Snijders BE, Huber M, van Ree R, Simoes-Wust AP, Dagnelie PC, van den Brandt PA. Fatty acids in breast milk and development of atopic eczema and allergic sensitisation in infancy. Allergy. 2011;66(1):5867. [PubMed] [Google Scholar]46.
Kesse-Guyot E, Baudry J, Assmann KE, Galan P, Hercberg S, Lairon D. Prospective association between consumption frequency of organic food and body weight change, risk of overweight or obesity: results from the NutriNet-Sant study. Br J Nutr. 2017;117(2):325334. [PubMed] [Google Scholar]47.
Baudry J, Mejean C, Peneau S, Galan P, Hercberg S, Lairon D, Kesse-Guyot E. Health and dietary traits of organic food consumers: results from the NutriNet-Sante study. Br J Nutr. 2015;114(12):20642073. [PubMed] [Google Scholar]48.
Alfano CM, Day JM, Katz ML, Herndon JE, 2nd, Bittoni MA, Oliveri JM, Donohue K, Paskett ED. Exercise and dietary change after diagnosis and cancer-related symptoms in long-term survivors of breast cancer: CALGB 79804. Psycho-Oncology. 2009;18(2):128133. [PMC free article] [PubMed] [Google Scholar]49.
Jacobs DR, Tapsell LC. Food synergy: the key to a healthy diet. Proc Nutr Soc. 2013;72(2):200206. [PubMed] [Google Scholar]50.
Olsson ME, Andersson CS, Oredsson S, Berglund RH, Gustavsson K-E. Antioxidant levels and inhibition of cancer cell proliferation in vitro by extracts from organically and conventionally cultivated strawberries. J Agric Food Chem. 2006;54(4):12481255. [PubMed] [Google Scholar]51.
Kazimierczak R, Hallmann E, Lipowski J, Drela N, Kowalik A, Pssa T, Matt D, Luik A, Gozdowski D, Rembiakowska E. Beetroot (Beta Vulgaris L.) and naturally fermented beetroot juices from organic and conventional production: metabolomics, antioxidant levels and anticancer activity. J Sci Food Agric. 2014;94(13):26182629. [PubMed] [Google Scholar]52.
Velimirov A, Huber M, Lauridsen C, Rembiakowska E, Seidel K, Bgel S. Feeding trials in organic food quality and health research. J Sci Food Agric. 2010;90(2):175182. [PubMed] [Google Scholar]53.
Huber M, van de Vijver LP, Parmentier H, Savelkoul H, Coulier L, Wopereis S, Verheij E, van der Greef J, Nierop D, Hoogenboom RA. Effects of organically and conventionally produced feed on biomarkers of health in a chicken model. Br J Nutr. 2010;103(5):663676. [PubMed] [Google Scholar]54.
Huber MAS, Coulier L, Wopereis S, Savelkoul H, Nierop D, Hoogenboom R. Enhanced catch-up growth after a challenge in animals on organic feed. Paris: International Conference on Nutrition & Growth; 2012. [Google Scholar]55.
Huber M, Knottnerus JA, Green L, Hvd H, Jadad AR, Kromhout D, Leonard B, Lorig K, Loureiro MI, JWMvd M, et al. How should we define health? BMJ. 2011;343 [PubMed]56.
Jensen MM, Jorgensen H, Halekoh U, Olesen JE, Lauridsen C. Can agricultural cultivation methods influence the healthfulness of crops for foods? J Agric Food Chem. 2012;60(25):63836390. [PubMed] [Google Scholar]57.
Srednicka-Tober D, Baranski M, Gromadzka-Ostrowska J, Skwarlo-Sonta K, Rembialkowska E, Hajslova J, Schulzova V, Cakmak I, Ozturk L, Krolikowski T, et al. Effect of crop protection and fertilization regimes used in organic and conventional production systems on feed composition and physiological parameters in rats. J Agric Food Chem. 2013;61(5):10171029. [PubMed] [Google Scholar]58.
Finamore A, Britti MS, Roselli M, Bellovino D, Gaetani S, Mengheri E. Novel approach for food safety evaluation. Results of a pilot experiment to evaluate organic and conventional foods. J Agric Food Chem. 2004;52(24):74257431. [PubMed] [Google Scholar]59.
Jensen MM, Halekoh U, Stokes CR, Lauridsen C. Effect of maternal intake of organically or conventionally produced feed on oral tolerance development in offspring rats. J Agric Food Chem. 2013;61(20):48314838. [PubMed] [Google Scholar]60.
Roselli M, Finamore A, Brasili E, Capuani G, Kristensen HL, Micheloni C, Mengheri E. Impact of organic and conventional carrots on intestinal and peripheral immunity. J Sci Food Agric. 2012;92(14):29132922. [PubMed] [Google Scholar]61.
van Bruggen AH, Gamliel A, Finckh MR. Plant disease management in organic farming systems. Pest Manag Sci. 2016;72(1):3044. [PubMed] [Google Scholar]62.
Zehnder G, Gurr GM, Kuhne S, Wade MR, Wratten SD, Wyss E. Arthropod pest management in organic crops. Annu Rev Entomol. 2007;52:5780. [PubMed] [Google Scholar]63.
Garibaldi LA, Carvalheiro LG, Vaissiere BE, Gemmill-Herren B, Hipolito J, Freitas BM, Ngo HT, Azzu N, Saez A, Astrom J, et al. Mutually beneficial pollinator diversity and crop yield outcomes in small and large farms. Science. 2016;351(6271):388391. [PubMed] [Google Scholar]64.
Gurr GM, Lu Z, Zheng X, Xu H, Zhu P, Chen G, Yao X, Cheng J, Zhu Z, Catindig JL, et al. Multi-country evidence that crop diversification promotes ecological intensification of agriculture. Nat Plants. 2016;2:16014. [PubMed] [Google Scholar]65. European Commission: COMMISSION REGULATION (EU) No 1107/2009 of 21 October 2009 concerning the placing of plant protection products on the market and repealing Council Directives 79/117/EEC and 91/414/EEC. In: Off J Eur Union 2009.
68.
Mallory EB, Halberg N, Andreasen L, Delate K, Ngouajio M. Innovations in organic food systems for sustainable production and ecosystem services: an introduction to the special issue of sustainable agriculture research. Sustain Agric Res. 2015;4(3):1. [Google Scholar]69. Beck A, Alexander C, Eduardo H, Anna Maria K, Koopmans J, Micheloni C, Moeskops C, Niggli B, Urs P, Ilse A, Susanne and Rasmussen. TP Organics: Strategic research and innovation agenda for organic food and farming. Belgium. 2014.
70.
International Centre for Research in Organic Food Systems (ICROFS) kologiens bidrag til samfundsgoder (the contribution of organic farming to public goods in Denmark, in Danish) 2015. [Google Scholar]71. Gustaf Forsberg: Control of cereal seed-borne diseases by hot humid air seed treatment, vol. 443; 2004.
72. Forsberg G. Control of cereal seed-borne diseases by hot humid air seed treatment. Diss. Swedish university of Agricultural Sciences, SLU. Uppsala, Sweden. 2003;2003:1305. ISBN 91-576-6496-X.
74.
European Food Safety Authority. The 2013 European Union Report on Pesticide Residues in Food. EFSA Journal. 2015;13:3. [PMC free article] [PubMed]75. European Food Safety Agency. The 2014 European Union report on pesticide residues in food. EFSA J. 2015;13(3):4038.
77.
Kortenkamp A, Backhaus T, Faust M. State of the art report on mixture toxicity. In., vol. study 070307/2007/485103/ETU/D.1. European Commission: Brussels; 2009. [Google Scholar]78. Beckman K: Exponering fr resthalter av pesticider i konventionellt odlade frukter, br och grnsaker inom EU och i tredje land jmfrt med konventionellt odlade i Sverige samt ekologiskt odlade. (Exposure for pesticide residues in conventionally grown fruits, berries and vegetables from the EU and third countries, compared to conventionally grown products from Sweden and to organically grown products, in Swedish). Bachelor thesis. 2015.
79.
European Commission, Directorate-General for Health and Food Safety . Final report of an audit carried out in Germany from 07 September 2015 to 11 September 2015 in order to evaluate pesticide residue controls in organic production. 2015. [Google Scholar]80.
CDC . Fourth National Report on human exposure to environmental chemicals, opdated tables september 2013. Washington: Centers for Disease Control and Prevention (CDC); 2013. [Google Scholar]81.
Viel JF, Warembourg C, Le Maner-Idrissi G, Lacroix A, Limon G, Rouget F, Monfort C, Durand G, Cordier S, Chevrier C. Pyrethroid insecticide exposure and cognitive developmental disabilities in children: the PELAGIE mother-child cohort. Environ Int. 2015;82:6975. [PubMed] [Google Scholar]82.
Cartier C, Warembourg C, Le Maner-Idrissi G, Lacroix A, Rouget F, Monfort C, Limon G, Durand G, Saint-Amour D, Cordier S, et al. Organophosphate insecticide metabolites in prenatal and childhood urine samples and intelligence scores at 6 years of age: results from the mother-child PELAGIE cohort (France) Environ Health Perspect. 2016;124(5):674680. [PMC free article] [PubMed] [Google Scholar]83.
Frry N, Guldner L, Saoudi A, Garnier R, Zeghnoun A, Bibondo M. Exposure of the French population to environmental chemicals. Volume 2 - Polychlorobiphenyls (NDL-PCBs) and pesticides, in French. Saint-Maurice: Institut de veille sanitaire; 2013. Exposition de la population franaise aux substances chimiques de l'environnement. Polychlorobiphnyles (PCB-NDL) et pesticides. [Google Scholar]84.
Heudorf U, Butte W, Schulz C, Angerer J. Reference values for metabolites of pyrethroid and organophosphorous insecticides in urine for human biomonitoring in environmental medicine. Int J Hyg Environ Health. 2006;209(3):293299. [PubMed] [Google Scholar]85.
Spaan S, Pronk A, Koch HM, Jusko TA, Jaddoe VW, Shaw PA, Tiemeier HM, Hofman A, Pierik FH, Longnecker MP. Reliability of concentrations of organophosphate pesticide metabolites in serial urine specimens from pregnancy in the generation R study. J Expo Sci Environ Epidemiol. 2015;25(3):286294. [PMC free article] [PubMed] [Google Scholar]86.
Roca M, Miralles-Marco A, Ferre J, Perez R, Yusa V. Biomonitoring exposure assessment to contemporary pesticides in a school children population of Spain. Environ Res. 2014;131C:7785. [PubMed] [Google Scholar]87.
Croes K, Den Hond E, Bruckers L, Govarts E, Schoeters G, Covaci A, Loots I, Morrens B, Nelen V, Sioen I, et al. Endocrine actions of pesticides measured in the Flemish environment and health studies (FLEHS I and II) Environ Sci Pollut Res Int. 2015;22(19):1458914599. [PubMed] [Google Scholar]88.
Wielgomas B, Nahorski W, Czarnowski W. Urinary concentrations of pyrethroid metabolites in the convenience sample of an urban population of northern Poland. Int J Hyg Environ Health. 2013;216(3):295300. [PubMed] [Google Scholar]89. Mrck T, Andersen H, Knudsen L: Organophosphate metabolites in urine samples from Danish children and women - measured in the Danish DEMOCOPHES population. Danish Environmental Protection Agency. 2017.
90. Tyler CR, Beresford N, van der Woning M, Sumpter JP, Thorpe K. Metabolism and environmental degradation of pyrethroid insecticides produce compounds with endocrine activities. Environ Toxicol Chem. Copenhagen. 2000;19(4):8019.
91.
Lu C, Toepel K, Irish R, Fenske RA, Barr DB, Bravo R. Organic diets significantly lower Childrens dietary exposure to Organophosphorus pesticides. Environ Health Perspect. 2006;114(2):260263. [PMC free article] [PubMed] [Google Scholar]92.
Oates L, Cohen M, Braun L, Schembri A, Taskova R. Reduction in urinary organophosphate pesticide metabolites in adults after a week-long organic diet. Environ Res. 2014;132(0):105111. [PubMed] [Google Scholar]93.
Bradman A, Quiros-Alcala L, Castorina R, Aguilar Schall R, Camacho J, Holland NT, Barr DB, Eskenazi B. Effect of organic diet intervention on pesticide exposures in young children living in low-income urban and agricultural communities. Environ Health Perspect. 2015;123(10):10861093. [PMC free article] [PubMed] [Google Scholar]94.
Ye M, Beach J, Martin JW, Senthilselvan A. Associations between dietary factors and urinary concentrations of organophosphate and pyrethroid metabolites in a Canadian general population. Int J Hyg Environ Health. 2015;218(7):616626. [PubMed] [Google Scholar]95.
Curl CL, Beresford SA, Fenske RA, Fitzpatrick AL, Lu C, Nettleton JA, Kaufman JD. Estimating pesticide exposure from dietary intake and organic food choices: the multi-ethnic study of atherosclerosis (MESA) Environ Health Perspect. 2015;123(5):475483. [PMC free article] [PubMed] [Google Scholar]96.
Goodson WH, 3rd, Lowe L, Carpenter DO, Gilbertson M, Manaf Ali A, Lopez de Cerain Salsamendi A, Lasfar A, Carnero A, Azqueta A, Amedei A, et al. Assessing the carcinogenic potential of low-dose exposures to chemical mixtures in the environment: the challenge ahead. Carcinogenesis. 2015;36(Suppl 1):S254S296. [PMC free article] [PubMed] [Google Scholar]97.
Kortenkamp A. Low dose mixture effects of endocrine disrupters and their implications for regulatory thresholds in chemical risk assessment. Curr Opin Pharmacol. 2014;19:105111. [PubMed] [Google Scholar]98.
Jacobsen PR, Axelstad M, Boberg J, Isling LK, Christiansen S, Mandrup KR, Berthelsen LO, Vinggaard AM, Hass U. Persistent developmental toxicity in rat offspring after low dose exposure to a mixture of endocrine disrupting pesticides. Reprod Toxicol. 2012;34(2):237250. [PubMed] [Google Scholar]99.
Bjorling-Poulsen M, Andersen HR, Grandjean P. Potential developmental neurotoxicity of pesticides used in Europe. Environ Health. 2008;7:50. [PMC free article] [PubMed] [Google Scholar]100.
Beronius A, Johansson N, Rudn C, Hanberg A. The influence of study design and sex-differences on results from developmental neurotoxicity studies of bisphenol a, implications for toxicity testing. Toxicology. 2013;311(12):1326. [PubMed] [Google Scholar]101.
Tweedale T, Lysimachou A, Muilerman H. Missed & Dismissed - pesticide regulators ignore the legal obligation to use independent science for deriving safe exposure levels. Brussels: PAN Europe; 2014. [Google Scholar]103.
Chiu YH, Gaskins AJ, Williams PL, Mendiola J, Jorgensen N, Levine H, Hauser R, Swan SH, Chavarro JE, European Ombudsman. Intake of fruits and vegetables with low-to-moderate pesticide residues is positively associated with semen-quality parameters among young healthy men. J Nutr. 2016;146(5):108492. [PMC free article] [PubMed]104.
Choi AL, Cordier S, Weihe P, Grandjean P. Negative confounding in the evaluation of toxicity: the case of methylmercury in fish and seafood. Crit Rev Toxicol. 2008;38(10):877893. [PMC free article] [PubMed] [Google Scholar]105. Ntzani EE, Chondrogiorgi M, Ntritsos G, Evangelou E, Tzoulaki I: Literature review on epidemiological studies linking exposure to pesticides and health effects. EFSA Supporting Publication. 2013:159.
106.
Moisan F, Spinosi J, Delabre L, Gourlet V, Mazurie JL, Benatru I, Goldberg M, Weisskopf MG, Imbernon E, Tzourio C, et al. Association of Parkinson's disease and its subtypes with agricultural pesticide exposures in men: a case-control study in France. EFSA Supporting Publication. 2013;10(10):EN497. 159 pp. [PMC free article] [PubMed]107.
Van Maele-Fabry G, Hoet P, Vilain F, Lison D. Occupational exposure to pesticides and Parkinson's disease: a systematic review and meta-analysis of cohort studies. Environ Int. 2012;46:3043. [PubMed] [Google Scholar]108.
Starling AP, Umbach DM, Kamel F, Long S, Sandler DP, Hoppin JA. Pesticide use and incident diabetes among wives of farmers in the agricultural health study. Occup Environ Med. 2014;71(9):629635. [PMC free article] [PubMed] [Google Scholar]109.
Dyck R, Karunanayake C, Pahwa P, Hagel L, Lawson J, Rennie D, Dosman J. Prevalence, risk factors and co-morbidities of diabetes among adults in rural Saskatchewan: the influence of farm residence and agriculture-related exposures. BMC Public Health. 2013;13:7. [PMC free article] [PubMed] [Google Scholar]110.
Schinasi L, Leon ME. Non-Hodgkin lymphoma and occupational exposure to agricultural pesticide chemical groups and active ingredients: a systematic review and meta-analysis. Int J Environ Res Public Health. 2014;11(4):44494527. [PMC free article] [PubMed] [Google Scholar]111.
Van Maele-Fabry G, Hoet P, Lison D. Parental occupational exposure to pesticides as risk factor for brain tumors in children and young adults: a systematic review and meta-analysis. Environ Int. 2013;56:1931. [PubMed] [Google Scholar]112.
Van Maele-Fabry G, Lantin AC, Hoet P, Lison D. Residential exposure to pesticides and childhood leukaemia: a systematic review and meta-analysis. Environ Int. 2011;37(1):280291. [PubMed] [Google Scholar]113.
Chen M, Chang CH, Tao L, Lu C. Residential exposure to pesticide during childhood and childhood cancers: a meta-analysis. Pediatrics. 2015;136(4):719729. [PubMed] [Google Scholar]114.
Andersen HR, Schmidt IM, Grandjean P, Jensen TK, Budtz-Jorgensen E, Kjaerstad MB, Baelum J, Nielsen JB, Skakkebaek NE, Main KM. Impaired reproductive development in sons of women occupationally exposed to pesticides during pregnancy. Environ Health Perspect. 2008;116(4):566572. [PMC free article] [PubMed] [Google Scholar]115.
Andersen HR, Debes F, Wohlfahrt-Veje C, Murata K, Grandjean P. Occupational pesticide exposure in early pregnancy associated with sex-specific neurobehavioral deficits in the children at school age. Neurotoxicol Teratol. 2015;47:19. [PubMed] [Google Scholar]116.
Wohlfahrt-Veje C, Andersen HR, Schmidt IM, Aksglaede L, Sorensen K, Juul A, Jensen TK, Grandjean P, Skakkebaek NE, Main KM. Early breast development in girls after prenatal exposure to non-persistent pesticides. Int J Androl. 2012;35(3):273282. [PubMed] [Google Scholar]117.
Wohlfahrt-Veje C, Andersen HR, Jensen TK, Grandjean P, Skakkebaek NE, Main KM. Smaller genitals at school age in boys whose mothers were exposed to non-persistent pesticides in early pregnancy. Int J Androl. 2012;35(3):265272. [PubMed] [Google Scholar]118.
Wohlfahrt-Veje C, Main KM, Schmidt IM, Boas M, Jensen TK, Grandjean P, Skakkebaek NE, Andersen HR. Lower birth weight and increased body fat at school age in children prenatally exposed to modern pesticides: a prospective study. Environ Health. 2011;10:79. [PMC free article] [PubMed] [Google Scholar]119.
Grandjean P, Landrigan PJ. Developmental neurotoxicity of industrial chemicals. Lancet. 2006;368(9553):21672178. [PubMed] [Google Scholar]120.
Young JG, Eskenazi B, Gladstone EA, Bradman A, Pedersen L, Johnson C, Barr DB, Furlong CE, Holland NT. Association between in utero organophosphate pesticide exposure and abnormal reflexes in neonates. Neurotoxicology. 2005;26(2):199209. [PubMed] [Google Scholar]121.
Eskenazi B, Marks AR, Bradman A, Harley K, Barr DB, Johnson C, Morga N, Jewell NP. Organophosphate pesticide exposure and neurodevelopment in young Mexican-American children. Environ Health Perspect. 2007;115(5):792798. [PMC free article] [PubMed] [Google Scholar]122.
Marks AR, Harley K, Bradman A, Kogut K, Barr DB, Johnson C, Calderon N, Eskenazi B. Organophosphate pesticide exposure and attention in young Mexican-American children: the CHAMACOS study. Environ Health Perspect. 2010;118(12):17681774. [PMC free article] [PubMed] [Google Scholar]123.
Bouchard MF, Chevrier J, Harley KG, Kogut K, Vedar M, Calderon N, Trujillo C, Johnson C, Bradman A, Barr DB, et al. Prenatal exposure to organophosphate pesticides and IQ in 7-year-old children. Environ Health Perspect. 2011;119(8):11891195. [PMC free article] [PubMed] [Google Scholar]124.
Engel SM, Wetmur J, Chen J, Zhu C, Barr DB, Canfield RL, Wolff MS. Prenatal exposure to organophosphates, paraoxonase 1, and cognitive development in childhood. Environ Health Perspect. 2011;119(8):11821188. [PMC free article] [PubMed] [Google Scholar]125.
Rauh VA, Garfinkel R, Perera FP, Andrews HF, Hoepner L, Barr DB, Whitehead R, Tang D, Whyatt RW. Impact of prenatal chlorpyrifos exposure on neurodevelopment in the first 3 years of life among inner-city children. Pediatrics. 2006;118(6):e1845e1859. [PMC free article] [PubMed] [Google Scholar]126.
Rauh V, Arunajadai S, Horton M, Perera F, Hoepner L, Barr DB, Whyatt R. Seven-year neurodevelopmental scores and prenatal exposure to chlorpyrifos, a common agricultural pesticide. Environ Health Perspect. 2011;119(8):11961201. [PMC free article] [PubMed] [Google Scholar]127.
Rauh VA, Perera FP, Horton MK, Whyatt RM, Bansal R, Hao X, Liu J, Barr DB, Slotkin TA, Peterson BS. Brain anomalies in children exposed prenatally to a common organophosphate pesticide. Proc Natl Acad Sci U S A. 2012;109(20):78717876. [PMC free article] [PubMed] [Google Scholar]128.
Rauh VA, Garcia WE, Whyatt RM, Horton MK, Barr DB, Louis ED. Prenatal exposure to the organophosphate pesticide chlorpyrifos and childhood tremor. Neurotoxicology. 2015;51:8086. [PMC free article] [PubMed] [Google Scholar]130.
Gonzalez-Alzaga B, Lacasana M, Aguilar-Garduno C, Rodriguez-Barranco M, Ballester F, Rebagliato M, Hernandez AF. A systematic review of neurodevelopmental effects of prenatal and postnatal organophosphate pesticide exposure. Toxicol Lett. 2014;230(2):104121. [PubMed] [Google Scholar]131.
Ross SM, McManus IC, Harrison V, Mason O. Neurobehavioral problems following low-level exposure to organophosphate pesticides: a systematic and meta-analytic review. Crit Rev Toxicol. 2013;43(1):2144. [PubMed] [Google Scholar]132.
Munoz-Quezada MT, Lucero BA, Barr DB, Steenland K, Levy K, Ryan PB, Iglesias V, Alvarado S, Concha C, Rojas E, et al. Neurodevelopmental effects in children associated with exposure to organophosphate pesticides: a systematic review. Neurotoxicology. 2013;39C:158168. [PMC free article] [PubMed] [Google Scholar]133.
Bouchard MF, Bellinger DC, Wright RO, Weisskopf MG. Attention-deficit/hyperactivity disorder and urinary metabolites of organophosphate pesticides. Pediatrics. 2010;125(6):e1270e1277. [PMC free article] [PubMed] [Google Scholar]134.
Wagner-Schuman M, Richardson JR, Auinger P, Braun JM, Lanphear BP, Epstein JN, Yolton K, Froehlich TE. Association of pyrethroid pesticide exposure with attention-deficit/hyperactivity disorder in a nationally representative sample of U.S. children. Environ Health. 2015;14(1):44. [PMC free article] [PubMed] [Google Scholar]135.
Quiros-Alcala L, Mehta S, Eskenazi B. Pyrethroid pesticide exposure and parental report of learning disability and attention deficit/hyperactivity disorder in U.S. children: NHANES 1999-2002. Environ Health Perspect. 2014;122(12):13361342. [PMC free article] [PubMed] [Google Scholar]136.
Oulhote Y, Bouchard MF. Urinary metabolites of organophosphate and Pyrethroid pesticides and behavioral problems in Canadian children. Environ Health Perspect. 2013;121(1112):13781384. [PMC free article] [PubMed] [Google Scholar]137.
Viel JF, Rouget F, Warembourg C, Monfort C, Limon G, Cordier S, Chevrier C: Behavioural disorders in 6-year-old children and pyrethroid insecticide exposure: the PELAGIE mother-child cohort. Occup Environ Med 2017. [PubMed]138.
Yolton K, Xu Y, Sucharew H, Succop P, Altaye M, Popelar A, Montesano MA, Calafat AM, Khoury JC. Impact of low-level gestational exposure to organophosphate pesticides on neurobehavior in early infancy: a prospective study. Environ Health. 2013;12(1):79. [PMC free article] [PubMed] [Google Scholar]139.
McKelvey W, Jacobson JB, Kass D, Barr DB, Davis M, Calafat AM, Aldous KM: Population-based biomonitoring of exposure to organophosphate and Pyrethroid pesticides in new York City. Environ Health Perspect. 2013. [PMC free article] [PubMed]140.
Bellanger M, Demeneix B, Grandjean P, Zoeller RT, Trasande L. Neurobehavioral Deficits, Diseases and Associated Costs of Exposure to Endocrine Disrupting Chemicals in the European Union. J Clin Endocrin Metab. 2015; 10.1210/jc.2014-4323. [PMC free article] [PubMed]141. European Food Safety Authority: Final addendum to the art. 21 review on chlorpyrifos - public version. 2014.
142.
Rapporteur Member State Spain. European Commission: Commission Regulation (EU) 2016/60 of 19 January 2016 amending Annexes II and III to Regulation (EC) No 396/2005 of the European Parliament and of the Council as regards maximum residue levels for chlorpyrifos in or on certain products. In: Off J Eur Union 2016. http://www.efsa.europa.eu/.143. European Food Safety Authority (EFSA). Refined risk assessment regarding certain maximum residue levels (MRLs) of concern for the active substance chlorpyrifos. 2015;13(6):4142.
144. European Food Safety Authority: Conclusion on the peer review of the pesticide human health risk assessment of the active substance chlorpyrifos. 2014;12(4):3640.
146.
Seufert V, Ramankutty N, Foley JA. Comparing the yields of organic and conventional agriculture. Nature. 2012;485(7397):229232. [PubMed] [Google Scholar]147.
van Huylenbroek G, Mondelaers K, Aertsens J, Mondelaers K, Aertsens J, van Huylenbroeck G. A meta-analysis of the differences in environmental impacts between organic and conventional farming. Br Food J. 2009;111(10):10981119. [Google Scholar]148.
Mie A, Laursen K, berg KM, Forshed J, Lindahl A, Thorup-Kristensen K, Olsson M, Knuthsen P, Larsen E, Husted S. Discrimination of conventional and organic white cabbage from a long-term field trial study using untargeted LC-MS-based metabolomics. Anal Bioanal Chem. 2014;406(12):28852897. [PMC free article] [PubMed] [Google Scholar]149.
Novotn H, Kmiecik O, Gazka M, Krtkov V, Hurajov A, Schulzov V, Hallmann E, Rembiakowska E, Hajlov J. Metabolomic fingerprinting employing DART-TOFMS for authentication of tomatoes and peppers from organic and conventional farming. Food Addit Contam Part A Chem Anal Control Expo Risk Assess. 2012;29(9):13351346. [PubMed] [Google Scholar]150.
Vallverd-Queralt A, Medina-Remn A, Casals-Ribes I, Amat M, Lamuela-Ravents RM. A Metabolomic approach differentiates between conventional and organic ketchups. J Agric Food Chem. 2011;59(21):1170311710. [PubMed] [Google Scholar]151.
Rhlig RM, Engel K-H. Influence of the input system (conventional versus organic farming) on metabolite profiles of maize (Zea Mays) kernels. J Agric Food Chem. 2010;58(5):30223030. [PubMed] [Google Scholar]152.
Chen P, Harnly JM, Lester GE. Flow injection mass spectral fingerprints demonstrate chemical differences in Rio red grapefruit with respect to year, harvest time, and conventional versus organic farming. J Agric Food Chem. 2010;58(8):45454553. [PMC free article] [PubMed] [Google Scholar]153.
Lehesranta SJ, Koistinen KM, Massat N, Davies HV, Shepherd LV, McNicol JW, Cakmak I, Cooper J, Luck L, Karenlampi SO, et al. Effects of agricultural production systems and their components on protein profiles of potato tubers. Proteomics. 2007;7(4):597604. [PubMed] [Google Scholar]154.
Nawrocki A, Thorup-Kristensen K, Jensen ON. Quantitative proteomics by 2DE and MALDI MS/MS uncover the effects of organic and conventional cropping methods on vegetable products. J Proteome. 2011;74(12):28102825. [PubMed] [Google Scholar]155.
Lu CG, Hawkesford MJ, Barraclough PB, Poulton PR, Wilson ID, Barker GL, Edwards KJ. Markedly different gene expression in wheat grown with organic or inorganic fertilizer. Proc R Soc Lond Ser BBiol Sci. 2005;272(1575):19011908. [PMC free article] [PubMed] [Google Scholar]156.
van Dijk JP, Cankar K, Hendriksen PJM, Beenen HG, Zhu M, Scheffer S, Shepherd LVT, Stewart D, Davies HV, Leifert C, et al. The identification and interpretation of differences in the Transcriptomes of organically and conventionally grown potato tubers. J Agric Food Chem. 2012;60(9):20902101. [PubMed] [Google Scholar]157.
Dangour AD, Dodhia SK, Hayter A, Allen E, Lock K, Uauy R. Nutritional quality of organic foods: a systematic review. Am J Clin Nutr. 2009;90(3):680685. [PubMed] [Google Scholar]158.
Brandt K, Leifert C, Sanderson R, Seal CJ. Agroecosystem management and nutritional quality of plant foods: the case of organic fruits and vegetables. Crit Rev Plant Sci. 2011;30(12):177197. [Google Scholar]159.
Baraski M, rednicka-Tober D, Volakakis N, Seal C, Sanderson R, Stewart GB, Benbrook C, Biavati B, Markellou E, Giotis C, et al. Higher antioxidant and lower cadmium concentrations and lower incidence of pesticide residues in organically grown crops: a systematic literature review and meta-analyses. Br J Nutr. 2014;112(05):794811. [PMC free article] [PubMed] [Google Scholar]160.
Bindraban PS, Dimkpa C, Nagarajan L, Roy A, Rabbinge R. Revisiting fertilisers and fertilisation strategies for improved nutrient uptake by plants. Biol Fertil Soils. 2015;51(8):897911. [Google Scholar]161.
Wiesler F. Marschner's mineral nutrition of higher plants. Third ed. San Diego: Academic Press; 2012. Nutrition and quality; pp. 271282. [Google Scholar]162.
Huber D, Rmheld V, Weinmann M. Relationship between nutrition, plant diseases and pests. In: Marschner P, editor. Marschners mineral nutrition of higher plants. third ed. 2012. pp. 283298. [Google Scholar]163.
Gsewell S. N:P ratios in terrestrial plants: variation and functional significance. New Phytol. 2004;164(2):243266. [PubMed] [Google Scholar]164.
Del Rio D, Rodriguez-Mateos A, Spencer JP, Tognolini M, Borges G, Crozier A. Dietary (poly)phenolics in human health: structures, bioavailability, and evidence of protective effects against chronic diseases. Antioxid Redox Signal. 2013;18(14):18181892. [PMC free article] [PubMed] [Google Scholar]165.
Treutter D. Managing phenol contents in crop plants by Phytochemical farming and breedingvisions and constraints. Int J Mol Sci. 2010;11(3):807857. [PMC free article] [PubMed] [Google Scholar]166.
Akesson A, Barregard L, Bergdahl IA, Nordberg GF, Nordberg M, Skerfving S. Non-renal effects and the risk assessment of environmental cadmium exposure. Environ Health Perspect. 2014;122(5):431438. [PMC free article] [PubMed] [Google Scholar]167.
Grant CA. Phosphate in Soils: Interaction with Micronutrients, Radionuclides and Heavy Metals. 2015. Influence of phosphate fertilizer on cadmium in agricultural soils and crops; p. 123. [Google Scholar]168.
EFSA Panel on Contaminants in the Food Chain Cadmium in food. Scientific opinion of the panel on contaminants in the food chain on a request from the European Commission on cadmium in food. EFSA J. 2009;980:1139. [Google Scholar]169.
de Mees C, Eduljee GH, Hutton M. Assessment and management of risks arising from exposure to cadmium in fertilisers. I. Sci Total Environ. 2002;291(13):167187. [PubMed] [Google Scholar]171.
Nziguheba G, Smolders E. Inputs of trace elements in agricultural soils via phosphate fertilizers in European countries. Sci Total Environ. 2008;390(1):5357. [PubMed] [Google Scholar]172.
Kratz S, Schnug E. Schwermetalle in P-Dngern (Heavy metals in P fertilisers, in German) Landbauforschung Vlkenrode Spec. 2005;286:3745. [Google Scholar]173. Baranski M, Steward G, Leifert C: Personal communication. 2016.
174.
Laursen KH, Schjoerring JK, Olesen JE, Askegaard M, Halekoh U, Husted S. Multielemental fingerprinting as a tool for authentication of organic wheat, barley, faba bean, and potato. J Agric Food Chem. 2011;59(9):43854396. [PubMed] [Google Scholar]175.
Gundersen V, Bechmann IE, Behrens A, Sturup S. Comparative investigation of concentrations of major and trace elements in organic and conventional Danish agricultural crops. 1. Onions (Allium Cepa Hysam) and peas (Pisum Sativum ping pong) J Agric Food Chem. 2000;48(12):60946102. [PubMed] [Google Scholar]176.
Jones K, Johnston A. Cadmium in cereal grain and herbage from long-term experimental plots at Rothamsted UK. Environ Pollut. 1989;57(3):199216. [PubMed] [Google Scholar]177.
Christensen BT, Elsgaard L. Handelsgdnings indflydelse p afgrders indhold af arsen, bly, cadmium, krom, kvikslv og nikkel (The influence of mineral fertilisers on the crops content of arsenic, lead, cadmium, chromium and nickel, in Danish) Tjele: DCA - Nationalt Center for Fdevarer og Jordbrug; 2013. [Google Scholar]178. Schnug E, Haneklaus N: Uranium in phosphate fertilizersreview and outlook. Uranium-past and future challenges. Cham: Springer; 2015;123130.
179.
Kratz S, Knappe F, Schnug E. Loads and fate of fertilizer-derived uranium Leiden: Backhuys. 2008. Uranium balances in agroecosystems; pp. 179190. [Google Scholar]180.
Bigalke M, Ulrich A, Rehmus A, Keller A. Accumulation of cadmium and uranium in arable soils in Switzerland. Environ Pollut. 2017;221:8593. [PubMed] [Google Scholar]181.
Liesch T, Hinrichsen S, Goldscheider N. Uranium in groundwater--fertilizers versus geogenic sources. Sci Total Environ. 2015;536:981995. [PubMed] [Google Scholar]182.
Birke M, Rauch U, Lorenz H. Uranium in stream and mineral water of the Federal Republic of Germany. Environ Geochem Health. 2009;31(6):693706. [PubMed] [Google Scholar]183.
Karlsson I, Friberg H, Steinberg C, Persson P. Fungicide effects on fungal community composition in the wheat phyllosphere. PLoS One. 2014;9(11):e111786. [PMC free article] [PubMed] [Google Scholar]184.
Karlsson I, Friberg H, Kolseth AK, Steinberg C, Persson P. Organic farming increases richness of fungal taxa in the wheat phyllosphere. Mol Ecol. 2017; [PubMed]185.
Bernhoft A, Torp M, Clasen PE, Loes AK, Kristoffersen AB. Influence of agronomic and climatic factors on Fusarium infestation and mycotoxin contamination of cereals in Norway. Food Addit Contam Part A Chem Anal Control Expo Risk Assess. 2012;29(7):11291140. [PMC free article] [PubMed] [Google Scholar]186.
Kabak B, Dobson AD, Var I. Strategies to prevent mycotoxin contamination of food and animal feed: a review. Crit Rev Food Sci Nutr. 2006;46(8):593619. [PubMed] [Google Scholar]187.
European Food Safety Authority Risks to human and animal health related to the presence of deoxynivalenol and its acetylated and modified forms in food and feed. EFSA J. 2017;15(9):4718. [PMC free article] [PubMed] [Google Scholar]188.
Warnecke S, Paulsen HM, Schulz F, Rahmann G. Greenhouse gas emissions from enteric fermentation and manure on organic and conventional dairy farmsan analysis based on farm network data. Org Agric. 2014;4(4):285293. [Google Scholar]189.
Palupi E, Jayanegara A, Ploeger A, Kahl J. Comparison of nutritional quality between conventional and organic dairy products: a meta-analysis. J Sci Food Agric. 2012;92(14):27742781. [PubMed] [Google Scholar]190.
Woods VB, Fearon AM. Dietary sources of unsaturated fatty acids for animals and their transfer into meat, milk and eggs: a review. Livest Sci. 2009;126(13):120. [Google Scholar]191.
Khiaosa-ard R, Kreuzer M, Leiber F. Apparent recovery of C18 polyunsaturated fatty acids from feed in cow milk: a meta-analysis of the importance of dietary fatty acids and feeding regimens in diets without fat supplementation. J Dairy Sci. 2015;98(9):63996414. [PubMed] [Google Scholar]192.
rednicka-Tober D, Baraski M, Seal CJ, Sanderson R, Benbrook C, Steinshamn H, Gromadzka-Ostrowska J, Rembiakowska E, Skwaro-Sota K, Eyre M. Higher PUFA and n-3 PUFA, conjugated linoleic acid, -tocopherol and iron, but lower iodine and selenium concentrations in organic milk: a systematic literature review and meta-and redundancy analyses. Br J Nutr. 2016:118. [PMC free article] [PubMed]193.
Schwendel BH, Wester TJ, Morel PCH, Tavendale MH, Deadman C, Shadbolt NM, Otter DE. Organic and conventionally produced milk - an evaluation of factors influencing milk composition. J Dairy Sci. 2015;98(2):721746. [PubMed] [Google Scholar]194.
Anderson KE. Comparison of fatty acid, cholesterol, and vitamin a and E composition in eggs from hens housed in conventional cage and range production facilities. Poult Sci. 2011;90(7):16001608. [PubMed] [Google Scholar]195.
Mugnai C, Sossidou EN, Dal Bosco A, Ruggeri S, Mattioli S, Castellini C. The effects of husbandry system on the grass intake and egg nutritive characteristics of laying hens. J Sci Food Agric. 2014;94(3):459467. [PubMed] [Google Scholar]196.
Rakonjac S, Bogosavljevi-Bokovi S, Pavlovski Z, krbi Z, Doskovi V, Petrovi M, Petrievi V. Laying hen rearing systems: a review of chemical composition and hygienic conditions of eggs. Worlds Poult Sci J. 2014;70(01):151164. [Google Scholar]197.
rednicka-Tober D, Baraski M, Seal C, Sanderson R, Benbrook C, Steinshamn H, Gromadzka-Ostrowska J, Rembiakowska E, Skwaro-Sota K, Eyre M, et al. Composition differences between organic and conventional meat: a systematic literature review and meta-analysis. Br J Nutr. 2016;115(06):9941011. [PMC free article] [PubMed] [Google Scholar]198. Mie A, Kesse-Guyot E, Kahl J, Rembiakowska E, Andersen HR, Grandjean P, Gunnarsson S: Human health implications of organic food and organic agriculture. www.europarl.europa.eu/RegData/etudes/STUD/2016/581922/EPRS_STU(2016)581922_EN.pdf. In. Edited by European Parliament - Parliamentary Research Services; 2016.
199.
Wanders AJ, Alssema M, de Koning EJP, le Cessie S, de Vries JH, Zock PL, Rosendaal FR, Md H, de Mutsert R. Fatty acid intake and its dietary sources in relation with markers of type 2 diabetes risk: the NEO study. Eur J Clin Nutr. 2017;71(2):245251. [PubMed] [Google Scholar]200.
van Valenberg HJF, Hettinga KA, Dijkstra J, Bovenhuis H, Feskens EJM. Concentrations of n-3 and n-6 fatty acids in Dutch bovine milk fat and their contribution to human dietary intake. J Dairy Sci. 2013;96(7):41734181. [PubMed] [Google Scholar]201.
Welch AA, Shakya-Shrestha S, Lentjes MA, Wareham NJ, Khaw KT. Dietary intake and status of n-3 polyunsaturated fatty acids in a population of fish-eating and non-fish-eating meat-eaters, vegetarians, and vegans and the product-precursor ratio [corrected] of alpha-linolenic acid to long-chain n-3 polyunsaturated fatty acids: results from the EPIC-Norfolk cohort. Am J Clin Nutr. 2010;92(5):10401051. [PubMed] [Google Scholar]202.
Astorg P, Arnault N, Czernichow S, Noisette N, Galan P, Hercberg S. Dietary intakes and food sources of n-6 and n-3 PUFA in French adult men and women. Lipids. 2004;39(6):527535. [PubMed] [Google Scholar]203.
EFSA Panel on Dietetic Products, Nutrition and Allergies . Scientific opinion on dietary reference values for fats, including saturated fatty acids, polyunsaturated fatty acids, monounsaturated fatty acids, trans fatty acids, and cholesterol. 2010. [Google Scholar]204.
Jakobsen MU, O'Reilly EJ, Heitmann BL, Pereira MA, Balter K, Fraser GE, Goldbourt U, Hallmans G, Knekt P, Liu S, et al. Major types of dietary fat and risk of coronary heart disease: a pooled analysis of 11 cohort studies. Am J Clin Nutr. 2009;89(5):14251432. [PMC free article] [PubMed] [Google Scholar]205.
Chen M, Li Y, Sun Q, Pan A, Manson JE, Rexrode KM, Willett WC, Rimm EB, Hu FB. Dairy fat and risk of cardiovascular disease in 3 cohorts of US adults. Am J Clin Nutr. 2016;104(5):12091217. [PMC free article] [PubMed] [Google Scholar]206.
Wang DD, Li Y, Chiuve SE, Stampfer MJ, Manson JE, Rimm EB, Willett WC, Hu FB. Association of Specific Dietary Fats with Total and Cause-Specific Mortality. JAMA Intern Med. 2016;176(8):11341145. [PMC free article] [PubMed] [Google Scholar]207.
de Souza RJ, Mente A, Maroleanu A, Cozma AI, Ha V, Kishibe T, Uleryk E, Budylowski P, Schunemann H, Beyene J, et al. Intake of saturated and trans unsaturated fatty acids and risk of all cause mortality, cardiovascular disease, and type 2 diabetes: systematic review and meta-analysis of observational studies. BMJ. 2015;351:h3978. [PMC free article] [PubMed] [Google Scholar]208.
Gayet-Boyer C, Tenenhaus-Aziza F, Prunet C, Marmonier C, Malpuech-Brugere C, Lamarche B, Chardigny JM. Is there a linear relationship between the dose of ruminant trans-fatty acids and cardiovascular risk markers in healthy subjects: results from a systematic review and meta-regression of randomised clinical trials. Br J Nutr. 2014;112(12):19141922. [PMC free article] [PubMed] [Google Scholar]209.
Lee SY, Pearce EN. Reproductive endocrinology: iodine intake in pregnancy--even a little excess is too much. Nat Rev Endocrinol. 2015;11(5):260261. [PMC free article] [PubMed] [Google Scholar]211.
Gartner R. Recent data on iodine intake in Germany and Europe. J Trace Elem Med Biol. 2016;37:8589. [PubMed] [Google Scholar]212.
Aburto N, Abudou M, Candeias V, Wu T, Organization WH . Effect and safety of salt iodization to prevent iodine deficiency disorders: a systematic review with meta-analyses. Geneva: World Health Organization; 2014. [Google Scholar]213.
Woolhouse M, Ward M, van Bunnik B, Farrar J. Antimicrobial resistance in humans, livestock and the wider environment. Philos Tran R Soc Lond B Biol Sci. 2015;370(1670):20140083. [PMC free article] [PubMed] [Google Scholar]214.
Silbergeld EK, Graham J, Price LB. Industrial food animal production, antimicrobial resistance, and human health. Annu Rev Public Health. 2008;29:151169. [PubMed] [Google Scholar]215.
Cully M. Public health: the politics of antibiotics. Nature. 2014;509(7498):S16S17. [PubMed] [Google Scholar]216.
European Food Safety Authority (EFSA) ECDC/EFSA/EMA second joint report on the integrated analysis of the consumption of antimicrobial agents and occurrence of antimicrobial resistance in bacteria from humans and food-producing animals. EFSA J. 2017;15(7):4872. [PMC free article] [PubMed] [Google Scholar]217.
WHO . Strategic and technical advisory group on antimicrobial resistance (STAG-AMR): report of the fifth meeting, 2324 November 2015, WHO Headquarters. Geneva: World Health Organization; 2016. p. 9. [Google Scholar]218.
Laxminarayan R, Duse A, Wattal C, Zaidi AKM, Wertheim HFL, Sumpradit N, Vlieghe E, Hara GL, Gould IM, Goossens H, et al. Antibiotic resistancethe need for global solutions. Lancet Infect Dis. 2013;13(12):10571098. [PubMed] [Google Scholar]219.
Martinez JL. Environmental pollution by antibiotics and by antibiotic resistance determinants. Environ Pollut. 2009;157(11):28932902. [PubMed] [Google Scholar]220.
Casewell M, Friis C, Marco E, McMullin P, Phillips I. The European ban on growth-promoting antibiotics and emerging consequences for human and animal health. J Antimicrob Chemother. 2003;52(2):159161. [PubMed] [Google Scholar]221.
Hao H, Cheng G, Iqbal Z, Ai X, Hussain HI, Huang L, Dai M, Wang Y, Liu Z, Yuan Z. Benefits and risks of antimicrobial use in food-producing animals. Front Microbiol. 2014;5:288. [PMC free article] [PubMed] [Google Scholar]222.
Mather AE, Reid SWJ, Maskell DJ, Parkhill J, Fookes MC, Harris SR, Brown DJ, Coia JE, Mulvey MR, Gilmour MW, et al. Distinguishable epidemics of multidrug-resistant salmonella Typhimurium DT104 in different hosts. Science. 2013;341(6153):15141517. [PMC free article] [PubMed] [Google Scholar]223.
Mather AE, Matthews L, Mellor DJ, Reeve R, Denwood MJ, Boerlin P, Reid-Smith RJ, Brown DJ, Coia JE, Browning LM, et al. An ecological approach to assessing the epidemiology of antimicrobial resistance in animal and human populations. Proc R Soc Lond B Biol Sci. 2012;279(1733):16301639. [PMC free article] [PubMed] [Google Scholar]224.
Bruckmeier K, Prutzer M. Swedish pig producers and their perspectives on animal welfare: a case study. Br Food J. 2007;109(11):906918. [Google Scholar]225.
Andreasen CB, Spickler AR, Jones BE. Swedish animal welfare regulations and their impact on food animal production. J Am Vet Med A. 2005;227(1):3440. [PubMed] [Google Scholar]226.
European Food Safety Authority Scientific opinion of the panel of animal health and welfare on the request from the Commission on the welfare of weaners and rearing pigs: effects of different space allowances and floor types. EFSA J. 2005;268:119. [Google Scholar]227.
European Food Safety Authority Scientific opinion of the panel of animal health and welfare on the request from the Commission on animal health and welfare in fattening pigs in relation to housing and husbandry. EFSA Journal. 2007;564:114. [Google Scholar]228.
Kijlstra A, Eijck IAJM. Animal health in organic livestock production systems: a review. Wagening J Life Sci. 2006;54(1):7794. [Google Scholar]229.
Hegelund L. Sundhed og medicinforbrug hos kologiske og konventionelle slagtesvin. 2006. Medicinforbrug og ddelighed i kologisk og konventionel slagtesvineproduktion (use of pharmaceuticals and mortality in organic and conventional pig production, in Danish) pp. 1316. [Google Scholar]230.
Wingstrand A, Struve T, Lundsby K, Vigre H, Emborg HD, Srensen AIV, Jensen VF. Fremtidens fdevaresikkerhed- Nye veje mod sikrere kd i Danmark. Denmark: Center for Bioetik og Risikovurdering; 2010. Antibiotikaresistens og -forbrug i slagtesvineproduktionen (antibiotic resistance and use in pig production, in Danish) pp. 98106. [Google Scholar]231.
Bennedsgaard TW, Klaas IC, Vaarst M. Reducing use of antimicrobials - experiences from an intervention study in organic dairy herds in Denmark. Livest Sci. 2010;131:183192. [Google Scholar]232.
Kuipers A, Koops W, Wemmenhove H. Antibiotic use in dairy herds in the Netherlands from 2005 to 2012. J Dairy Sci. 2016;99(2):16321648. [PubMed] [Google Scholar]233.
Fall N, Emanuelson U. Milk yield, udder health and reproductive performance in Swedish organic and conventional dairy herds. J Dairy Res. 2009;76(4):402410. [PubMed] [Google Scholar]235.
Snary E, Pleydell E, Munday D. Investigation of persistence of antimicrobial resistant organisms in broiler flocks: a mathematical model. UK: Veterinary Laboratories Agency; 2006. [Google Scholar]236.
Von Borell E, Srensen JT. Organic livestock production in Europe: aims, rules and trends with special emphasis on animal health and welfare. Livest Prod Sci. 2004;90(1):39. [Google Scholar]237.
Hemme M, van Rennings L, Hartmann M, von Mnchhausen C, Ksbohrer A, Kreienbrock L. Antibiotikaeinsatz in der Nutztierhaltung in Deutschland. Erste Ergebnisse zu zeitlichen Trends im wissenschaftlichen Projekt VetCAb-Sentinel Antibiotic use in livestock production in Germany. First results of temporal trends in the scientific project VetCAb-Sentinel (in German) Deutsches Tierrzteblatt. 2016;4:516520. [Google Scholar]239.
Persoons D, Dewulf J, Smet A, Herman L, Heyndrickx M, Martel A, Catry B, Butaye P, Haesebrouck F. Antimicrobial use in Belgian broiler production. Prev Vet Med. 2012;105(4):320325. [PubMed] [Google Scholar]240.
Woolhouse MEJ, Ward MJ. Sources of antimicrobial resistance. Science. 2013;341(6153):14601461. [PubMed] [Google Scholar]241.
European Food Safety Authority SCIENTIFIC REPORT OF ECDC, EFSA AND EMA ECDC/EFSA/EMA first joint report on the integrated analysis of the consumption of antimicrobial agents and occurrence of antimicrobial resistance in bacteria from humans and food-producing animals. EFSA Journal. 2015;13(1)(4006):114. [Google Scholar]242.
Kemper N. Veterinary antibiotics in the aquatic and terrestrial environment. Ecol Indic. 2008;8(1):113. [Google Scholar]243.
Aarestrup FM. Veterinary drug usage and antimicrobial resistance in bacteria of animal origin. Basic Clin Pharmacol Toxicol. 2005;96:271281. [PubMed] [Google Scholar]244.
sterberg J, Wingstrand A, Jensen AN, Kerouanton A, Cibin V, Barco L, Denis M, Aabo S, Bengtsson B. Antibiotic resistance in Escherichia coli from pigs in organic and conventional farming in four European countries. PloS one. 2016;11(6):e0157049. [PMC free article] [PubMed]245.
Sapkota AR, Kinney EL, George A, Hulet RM, Cruz-Cano R, Schwab KJ, Zhang G, Joseph SW. Lower prevalence of antibiotic-resistant salmonella on large-scale US conventional poultry farms that transitioned to organic practices. Sci Total Environ. 2014;476:387392. [PubMed] [Google Scholar]246.
Leverstein-van Hall MA, Dierikx CM, Stuart JC, Voets GM, van den Munckhof MP, van Essen-Zandbergen A, Platteel T, Fluit AC, van de Sande-Bruinsma N, Scharinga J, et al. Dutch patients, retail chicken meat and poultry share the same ESBL genes, plasmids and strains. Clin Microbiol Infec. 2011;17(6):873880. [PubMed] [Google Scholar]247.
de Neeling AJ, van den Broek MJM, Spalburg EC, van Santen-Verheuvel MG, Dam-Deisz WDC, Boshuizen HC, de Giessen AWV, van Duijkeren E, Huijsdens XW. High prevalence of methicillin resistant Staphylococcus Aureus in pigs. Vet Microbiol. 2007;122(34):366372. [PubMed] [Google Scholar]248.
Fromm S, Beiwanger E, Ksbohrer A, Tenhagen B-A. Risk factors for MRSA in fattening pig herds a meta-analysis using pooled data. Prev Vet Med. 2014;117(1):180188. [PubMed] [Google Scholar]249.
Aubry-Damon H, Grenet K, Sall-Ndiaye P, Che D, Cordeiro E, Bougnoux ME, Rigaud E, Le Strat Y, Lemanissier V, Armand-Lefevre L, et al. Antimicrobial resistance in commensal flora of pig farmers. Emerg Infect Dis. 2004;10(5):873879. [PMC free article] [PubMed] [Google Scholar]250.
Armand-Lefevre L, Ruimy R, Andremont A. Clonal comparison of Staphylococcus Aureus isolates from healthy pig farmers, human controls, and pigs. Emerg Infect Dis. 2005;11(5):711714. [PMC free article] [PubMed] [Google Scholar]251.
Gerzova L, Babak V, Sedlar K, Faldynova M, Videnska P, Cejkova D, Jensen AN, Denis M, Kerouanton A, Ricci A, et al. Characterization of antibiotic resistance gene abundance and microbiota composition in feces of organic and conventional pigs from four EU countries. PLoS One. 2015;10(7):e0132892. [PMC free article] [PubMed] [Google Scholar]252.
Osterberg J, Wingstrand A, Nygaard Jensen A, Kerouanton A, Cibin V, Barco L, Denis M, Aabo S, Bengtsson B. Antibiotic resistance in Escherichia Coli from pigs in organic and conventional farming in four European countries. PLoS One. 2016;11(6):e0157049. [PMC free article] [PubMed] [Google Scholar]253.
Gleeson BL, Collins AM. Under what conditions is it possible to produce pigs without using antimicrobials? Anim Prod Sci. 2015;55(12):14241431. [Google Scholar]254.
Tilman D, Clark M. Global diets link environmental sustainability and human health. Nature. 2014;515(7528):518522. [PubMed] [Google Scholar]256. Bourguet D, Guillemaud T: The hidden and external costs of pesticide use. In: Sustainable Agriculture Reviews. Cham: Springer; 2016;35120.
257.
Kahl J, Alborzi F, Beck A, Bugel S, Busscher N, Geier U, Matt D, Meischner T, Paoletti F, Pehme S, et al. Organic food processing: a framework for concept, starting definitions and evaluation. J Sci Food Agric. 2014;94(13):25822594. [PubMed] [Google Scholar]258.
Monteiro CA, Levy RB, Claro RM, de Castro IR, Cannon G. Increasing consumption of ultra-processed foods and likely impact on human health: evidence from Brazil. Public Health Nutr. 2011;14(1):513. [PubMed] [Google Scholar]259.
Fardet A, Rock E, Bassama J, Bohuon P, Prabhasankar P, Monteiro C, Moubarac JC, Achir N. Current food classifications in epidemiological studies do not enable solid nutritional recommendations for preventing diet-related chronic diseases: the impact of food processing. Adv Nutr (Bethesda, Md) 2015;6(6):629638. [PMC free article] [PubMed] [Google Scholar]261. European Parliament and Council of the European Union: Directive 2009/128/EC of the European Parliament and of the Council of 21 October 2009 establishing a framework for Community action to achieve the sustainable use of pesticides. Eur-Lex 2009.
262.
Sofi F, Macchi C, Abbate R, Gensini GF, Casini A. Mediterranean diet and health status: an updated meta-analysis and a proposal for a literature-based adherence score. Public Health Nutr. 2014;17(12):27692782. [PMC free article] [PubMed] [Google Scholar]263.
UNEP . Avoiding future famines: strengthening the ecological foundation of food security through sustainable food systems. 2012. [Google Scholar]265.
Sez-Almendros S, Obrador B, Bach-Faig A, Serra-Majem L. Environmental footprints of Mediterranean versus western dietary patterns: beyond the health benefits of the Mediterranean diet. Environ Health. 2013;12(1):118. [PMC free article] [PubMed] [Google Scholar]266.
Mithril C, Dragsted LO, Meyer C, Blauert E, Holt MK, Astrup A. Guidelines for the new Nordic diet. Public Health Nutr. 2012;15(10):19411947. [PubMed] [Google Scholar]267.
Mithril C, Dragsted LO, Meyer C, Tetens I, Biltoft-Jensen A, Astrup A. Dietary composition and nutrient content of the new Nordic diet. Public Health Nutr. 2013;16(05):777785. [PMC free article] [PubMed] [Google Scholar]268.
Saxe H. The new Nordic diet is an effective tool in environmental protection: it reduces the associated socioeconomic cost of diets. Am J Clin Nutr. 2014;99(5):11171125. [PubMed] [Google Scholar]269.
Poulsen SK, Due A, Jordy AB, Kiens B, Stark KD, Stender S, Holst C, Astrup A, Larsen TM. Health effect of the new Nordic diet in adults with increased waist circumference: a 6-mo randomized controlled trial. Am J Clin Nutr. 2014;99(1):3545. [PubMed] [Google Scholar]270.
Strassner C, Cavoski I, Di Cagno R, Kahl J, Kesse-Guyot E, Lairon D, Lampkin N, Loes AK, Matt D, Niggli U, et al. How the organic food system supports sustainable diets and translates these into practice. Front Nutr. 2015;2:19. [PMC free article] [PubMed] [Google Scholar]271.
Moomaw W, Griffin T, Kurczak K, Lomax J. United Nations Environment Programme, Tech Rep. 2012. The critical role of global food consumption patterns in achieving sustainable food systems and food for all. [Google Scholar]